126
Ray Pete: Phosphates and Aging
Excess phosphate It can lead to increased inflammation, tissue atrophy, blood vessel calcification, cancer, dementia and, generally speaking, all aging processes. This topic is becoming very important as the use of phosphates as food additives increases.
Previously, it was believed that complications of chronic kidney disease associated with elevated values of serum phosphates is a phenomenon characteristic of these diseases. But opening klotho (named after one of the heroes of Greek mythology), regulating the balance of phosphates, led to the need to rethink the biological role of phosphates.
In the 19th century, phosphorus was commonly referred to as brain food, and from about 1970 onwards, its involvement in cellular regulation was at the heart of reductionist thinking. ATP, adenosine triphosphate, is considered as an energy source that provides cellular movement, and also provides energy to the “pumps” that support the state of life, as a source of cyclic adenosine monophosphate, which is a common activator of cells, as a donor of the phosphate group, which activates a large number of proteins in the “phosphorylation cascade”.When tissues are calcified in the process of aging, the responsibility for this is assigned to calcium (while ignoring the existence of calcium phosphate crystals in tissues) and therefore low-calcium diets are recommended. More recently, calcium has been blamed for the fact that calcium supplements did not give the expected effect, despite the fact that in addition to calcium, other substances such as citrate, phosphate, orotate, aspartate and lactate are also present in these supplements.
I have a different view of the “phosphorylation cascade” and other cellular functions of phosphate; it was formed from my understanding of the role of water in cellular physiology. A popular view is that the stimulus changes the shape of the receptor protein, which forces it to work as an enzyme, catalyzing the transition of the phosphate group from ATP to another protein, which in turn changes its shape and translates into an active state, resulting in the transfer of phosphate groups to other molecules or the removal of phosphates from active enzymes in chain reactions. This is a standard biochemistry that can be replicated in a test tube.
Since about 1970, when it was already well known about the involvement of phosphorylation in the activation of enzymes in the breakdown of glycogen, scientists began to notice that when muscle cells are reduced, the enzyme glycogen phosphorylase is instantly activated, and then phosphorylation occurs. The activation of the enzyme involves phosphorylation, so if something else first activated the enzyme (by changing its shape), then the addition of a phosphate group cannot be considered the sole cause of this process in the usual reductionist sense. Phosphorylation is one of the participants in a complex causal process. I saw this as a possible example of the effect that a changing water structure has on protein structure and function.
As you know, if the temperature drops below a certain value, enzymes suddenly lose activity. This is because soluble proteins take on a form in which their hydrophobic elements, i.e. fat-like side chains of amino acids, end up inside, and parts of the chain with water-soluble amino acids are placed outside, coming into contact with water. The “humidity” of water, its desire to reject the oily parts of the protein molecule decreases as the temperature drops, so some proteins have an unstable state in which the relatively hydrophobic group is no longer repelled by the colder water surrounding it.
In a living cell, water is always very close to the surface of fats or fat-like proteins. In a series of experiments that began in the 1960s, Walter Drost-Hansen showed that regardless of the nature of matter, water near the surface changes structurally, becoming less dense, more bulky. This water is more “lipophilic”, it adapts to the presence of fatty material, as if it is colder. Changes in the properties of water also affect the solubility of ions, with potassium solubility increasing and solubility of sodium, magnesium and calcium falling (Wiggins, 1973).
When a muscle contractes, it decreases in volume (Abbott and Baskin, 1962). Muscles also contract under extremely high pressure. In both cases, the productive reduction process involves a slight decrease in volume. During the contraction of a muscle or nerve, heat is released, which leads to an increase in temperature. During rest, heat is absorbed during the recovery process (Curtin and Woledge, 1974; Westphal, et al., 1999; Constable, et al. 1997). In the case of a nerve, following the heating caused by excitation, the nerve temperature drops below the initial temperature (Abbot, et al., 1965). Muscle stretching leads to energy absorption (Constable, et al., 1997). Such energetic changes, unaccompanied by chemical changes, have led some researchers to conclude that the creation of muscle tension is a process "driven by entropy" (Davis and Rodgers, 1995).
In 1858, Kelvin wrote about the physics of water in a soap bubble: "... if a film, such as a soap bubble, is stretched, ... it cools ..." This description also applies to the behavior of nerves and muscles that absorb energy at the time of relaxation (or lengthening) and release it when excited / contracted.
Several groups of experimenters over the past 60 years have tried to figure out what happens to the missing heat; some have suggested the presence of an electrical or osmotic storage, and some have shown that ATP is generated when stretched, and have therefore advocated the idea of chemical storage. The possibility of physical storage in the form of structural changes in the water-protein-fat system interacting with chemical changes such as ATP synthesis has not been studied.
In early studies of muscle chemistry and muscle contraction, it was found that adding ATP to a viscous solution of muscle extract reduced its viscosity, and muscle loss of ATP led to its hardening, as in rigor. If the pH value was not too acidic, then with a decrease in the content of ATP in the dead muscle, its reduction occurred. St. Györdy found that the muscle hardened by rigor became soft again when ATP was added.
Major rigor is extreme fatigue or energy depletion. In early studies, the phenomenon of “fatigue contracture” was described, in which the muscle reduced to the loss of the ability to respond to stimulation is reduced as much as possible (this condition is also called delayed relaxation). Ischemic contracture in the absence of circulation occurs when muscle glycogen is exhausted, and thus the anaerobic mechanism of ATP production stops (Kingsley, et al., 1991). Delayed hypothyroid muscle relaxation is another situation in which ATP is clearly required to relax. (In the Achilles tendon reflex test for hypothyroidism, the relaxation rate is slowed down, which can be seen visually.) T-wave delay on the electrocardiogram, diastolic contracture in heart failure is still the same process of delayed relaxation. By introducing the active thyroid hormone, T3, it is possible to quickly return to normal the relaxation rate, such a beneficial effect of the hormone is used in heart failure (Pingitore, et al., 2008; Wang, et al., 2006; Pantos, et al., 2007; Galli, et al., 2008).
Most of the magnesium in the cells is associated with ATP, the magnesium-ATP complex is a factor in muscle relaxation. Lack of ATP or magnesium leads to muscle spasms. When a cell is stimulated, ATP releases not only inorganic phosphate, but also magnesium. At a pH of more than 6.7, phosphate is twice ionized, exerting the same structural effect on water as magnesium, calcium and sodium: water molecules are strongly attracted to the concentrated electric charge of the ion. The increase in the concentration of free phosphate and magnesium counteracts the effect of fat and protein surfaces on the structure of water, leads to a decrease in the solubility of potassium in water, and also increases the “lipophobic” tendency of water to minimize contact with fats and fat-like areas on the surface of proteins, forcing proteins to rearrange.
Observations concerning the interactions in muscles and nerves of water, dissolved substances, and proteins offer a clear context for understanding contraction and conduction processes that is not found in familiar descriptions based on the concepts of cell membranes, pumps, and crossed bridges. I think these observations also offer a uniquely useful context for understanding the possible dangerous effects of excess phosphate in the body.
Some scientists (M. Thomson, J. Gunawardena, A. K. Manrai) show that the principle of acting masses helps to simplify the understanding of phosphorylation and dephosphorylation networks that are involved in cellular regulation. Regardless of protein phosphorylation, the presence of a phosphate ion in cellular water changes the ion selectivity of the cell, shifting the balance towards increased sodium and calcium absorption, reducing potassium and showing a tendency to depolarize and “activate” the cell.
Approximately 99% of publications that discuss muscle contraction do not address the role of water; water is also ignored when discussing energy production in mitochondria. Failure to produce mitochondrial energy leads to lipid peroxidation, activates inflammatory processes and can cause disintegration of the energy-producing structure. Increased concentration of phosphate reduces mitochondrial energy production (Duan and Karmazyn, 1989), causes lipid peroxidation (Kowaltowski, et al., 1996), triggers inflammatory processes, enhances tissue atrophy, fibrosis and cancer.
For twenty years, it has been known that a metabolic problem leading to loss of calcium in bones causes calcium buildup in soft tissues, such as blood vessels. Until recently, it was believed that phosphates play a passive role in the formation of calcium phosphate crystals, but some specific “mechanistic” effects have been identified.
For example, an increased concentration of phosphate leads to an increase in the inflammatory cytokine, osteopontin (Fatherazi, et al., 2009), which is known for its ability to activate the decalcification process in the bones and to participate in calcification processes in the arteries (Tousoulis, et al., 2012). Phosphates increase calcification in the kidneys (Bois and Selye, 1956), and osteopontin, due to the activation of inflammatory T cells, is involved in the development of glomerulonephritis and inflammatory skin reactions (Yu, et al., 1998).
The high content of phosphates in the diet increases the concentration of serum osteopontin, as well as serum phosphate and parathormone, contributes to the formation of skin tumors (Camalier, et al., 2010). In addition to activating cells and cellular systems, phosphates (like other ions with a high charge-to-size ratio, including citrate) are capable of activating viruses (Yamanaka, et al., 1995; Gouvea, et al., 2006). Aromatase, an enzyme that synthesizes estrogen, is sensitive to phosphate concentrations (Bellino and Holben, 1989).
Generally speaking, increased dietary phosphate enhances the activity of an important regulatory enzyme, protein kinase B, which promotes organ growth. A diet high in phosphate leads to an increase in liver (Xu et al., 2008) and lungs (Jin et al., 2007), supports the growth of lung cancer (Jin et al., 2009).But drastically restricting phosphates will not be the right solution, as their deficiency stimulates phosphate transport in cells, increasing cellular uptake of phosphates and having a similar effect as in conditions of excess phosphate, that is, increasing the growth of lung cancer (Xu et al., 2010).. The optimal content of phosphates in the diet and their share in the mineral composition of nutrition have not yet been determined.
An increase in phosphate levels slows down mitochondrial energy production, and a decrease in the concentration of phosphates in the intercellular space leads to an increase in the respiratory index and the effectiveness of ATP formation. To the same effect leads the "deficiency" of polyunsaturated fatty acids (Nogueira et al., 2001) and the consumption of fructose (Green et al., 1993; Lu et al., 1994).
In 1938, an experiment was conducted (Brown et al.) on a man who for six months received a diet of 2500 calories per day, consisting of sucrose syrup, a gallon of milk (partly in the form of grain cottage cheese), the juice of half an orange, as well as certain vitamins and minerals in the form of additives.
This experiment was supposed to demonstrate the irreplaceability of unsaturated fats. The members of the experimental group were very puzzled that surprisingly they did not observe normal fatigue at the end of the working day. He normalized high blood pressure and high cholesterol, and completely stopped migraines, which he suffered all his life. He had an increase in respiratory coefficient (carbon dioxide production increased) and resting metabolic rate. I think the most interesting result of this experiment is the reduction of phosphate in the blood of the subject. He had a fasting blood test twice. The serum neo-limited phosphorus content was 3.43 and 2.64 mg per 100 ml of plasma. At the end of the study, he returned to his normal diet, and his blood phosphorus levels were 4.2 mg/100 ml six months later. It can be assumed that the decrease in phosphates in the body occurred both due to a deficiency of “essential” unsaturated fatty acids, and due to the intake of high doses of sucrose.
In 2000, convinced of the dangerous properties of fructose, researchers suggested that switching to a diet with a magnesium deficiency would increase the harm caused by fructose. And eleven people took part in a six-month experiment, during which they received a diet high in corn starch or syrup, rich in fructose. They were also given other typical American foods, either extremely low in magnesium or with some deficiency. The conclusion reached by the researchers was clearly spelled out in the title of their paper and was that the combination described above significantly worsens the mineral balance in the body.
However, in the context of other studies on the effects of fructose on phosphates, I do not believe the above conclusions are correct. Even in the case of the extremely magnesium-poor diet, the balance of both magnesium and calcium was positive, from which it can be concluded that on average the subjects’ bodies consumed very little calcium and magnesium, people aged 22 to 40 do not seem to grow very much.
With a stable intake of both calcium and magnesium, with a significant excess of the absorbable level of calcium, it can be assumed that the minerals were absorbed in the bones. However, in the case of a “high-fructose” diet, their phosphate balance was slightly negative. If sugar had the same effect as in the William Brown experiment in 1938 (and in animal experiments), a slight decrease in phosphate could be explained by a decrease in its amount in the blood and other fluids, but as the experiment continued, this phenomenon must at some stage reflect changes in bone composition. When body fluids contain more carbon dioxide, calcium carbonate can be deposited in bones (Messier et al., 1979). Increased carbon dioxide levels can lead to a long-term negative phosphate balance due to the role that carbon dioxide plays in bone biochemistry when interacting with calcium and magnesium.
Another important property of carbon dioxide is its ability to regulate both calcium and phosphate simultaneously, increasing calcium absorption and retention (Canzanello et al., 1995) and enhancing phosphate release. Increased concentrations of carbon dioxide (as dissolved gas) and bicarbonate (sodium bicarbonate) lead to increased release of phosphates in the urine, even in the absence of parathyroid hormone. If the concentration of serum bicarbonate is below normal values, the reabsorption of phosphates by the kidneys increases significantly (Jehle et al., 1999). Acetazolamide increases the retention of carbon dioxide by the body, increasing the excretion of phosphates in the urine. A large proportion of calcium in the blood is a positively charged complex consisting of calcium and bicarbonate, its charge is equal to one (Hughes et al., 1984). Failure to handle this complex form of calcium leads to errors both in determining the level of calcium in the blood and in interpreting its physiological effects, including its action in the intercellular space. Hyperventilation can lead skeletal muscles into a convulsive state, constrict coves, excite platelets and other cells; removing carbon dioxide from the blood reduces the concentration of carbonic acid, altering the state and function of calcium. Hyperventilation leads to the growth of phosphate and parathyroid hormone, reduces the calcium content (Krapf et al., 1992).
Since estrogen tends to cause hyperventilation, and thus a decrease in carbon dioxide concentration, its role in phosphate metabolism should be studied more carefully. In the work of Han et al. (2002) and Xu et al. (2003), it was shown that estrogen increases the reabsorption of phosphate by the kidneys, but estrogen also leads to an increase in cortisol, which reduces reabsorption, so the effect of estrogen on the whole body should be taken into account.
The solubilizing effect on calcium from bicarbonate, combined with its ability to phosphaturia, may be responsible for the relaxing effect of carbon dioxide on blood vessels and smooth bronchial muscles, and also underlies the mechanism of preventing vascular calcification by thyroid hormone (Sato et al., 2005, Tatar, 2009, Kim et al., 2012). The elasticity of blood vessels and the heart is enhanced by carbon dioxide and, on the contrary, reduced with hypothyroidism, heart failure and under the influence of phosphate.
Fructose not only reduces the concentration of phosphate in the intercellular space, but also reduces the amount of phosphate absorbed in the gastrointestinal tract from food (Kirchner et al., 2008), and Milne-Nielsen studies conclude that it also increases the loss of phosphate through the kidneys. The “anti-aging” protein klotho enhances the ability of the kidneys to excrete phosphate and, like fructose, it supports energy production and thermogenesis (Mori et al., 2000).
A decrease in phosphate in the blood leads to a decrease in parathyroid hormone. Parathormone, by preventing the reabsorption of phosphate by the kidneys, leads to the production of serotonin by mast cells (and serotonin increases the renal reabsorption of phosphate) and possibly has other anti-inflammatory properties. For example, deletion of the PTH gene compensates for the negative effects (accelerated calcification and osteoporosis) of deletion of the klotho gene by preventing the growth of osteopontin (Yuan et al., 2012).
Niacinamide is another nutrient that reduces serum phosphate (Cheng et al., 2008), suppressing its absorption in the intestine (Katai et al., 1989) and reducing its reabsorption in the kidneys (Campbell et al., 1989). It is possible that the ability of niacinamide to reduce the level of free fatty acids by inhibiting lipolysis may play a role in the process of its effect on phosphate (similar to the phosphatoponizing effect in the deficiency of polyunsaturated fatty acids). Aspirin is another anti-lipolytic (de Zentella et al., 2002) that stimulates energy production from sugar and reduces phosphate, possibly contributing to magnesium retention (Yamada and Morohashi, 1986).
A diet containing enough calcium to limit the activity of the parathyroid glands and low amounts of phosphate and polyunsaturated fats, which uses sugar rather than starch as the main source of carbohydrates, as well as possible supplements of aspirin and niacinamide, should contribute to the fight against degenerative processes associated with high levels of phosphate: weakness, heart failure, discoordination of movements, hypogonadism, infertility, vascular calcification, emphysema, cancer, osteosis, osteosis, osteosis, 2010, drosis, and skin drosis (Oki, 2012, drosis, and derazka, and derazka, and droska, droska, and droscia, drosis, and drosis, 2012);The foods most rich in phosphate relative to their calcium content are muesli, legumes, meat and fish. Phosphate is added to many semi-finished products. Foods with a higher, safer ratio of calcium to phosphate are cabbage leaves and beets, many fruits, milk and cheese. Coffee, being a good source of magnesium, seems to be very effective in reducing phosphate due to its antagonism to adenosine. (Coulson et al., 1991).
Although elevated phosphate usually leads to vascular calcification (increases their stiffness and, accordingly, increases systolic blood pressure), in the case when most of the phosphate comes from food from milk and cheese, epidemiologically its effect is associated with a decrease in blood pressure (Takeda et al., 2012).
The toxicity of phosphate leads to some very interesting conclusions regarding stress and aging and helps explain the protective effects of carbon dioxide, thyroid hormone, sugar, niacinamide and calcium. Its toxicity suggests that the effects of other natural foods used as food additives should be carefully studied. For example, excess citric acid can activate dormant cancer cells (Havard et al., 2011) and lead to their malignancy (Blüml et al., 2011).Generally speaking, research to establish optimal ratios between minerals, fats, amino acids and other substances in foods, as well as studying their interaction with natural toxins, antinutrients and substances that destroy the body’s hormonal system, has barely begun.published
© Ray Pete.
P.S. And remember, just changing our consumption – together we change the world!
Source: //ekiri22.blogspot.com/2015/02/blog-post.html
Previously, it was believed that complications of chronic kidney disease associated with elevated values of serum phosphates is a phenomenon characteristic of these diseases. But opening klotho (named after one of the heroes of Greek mythology), regulating the balance of phosphates, led to the need to rethink the biological role of phosphates.
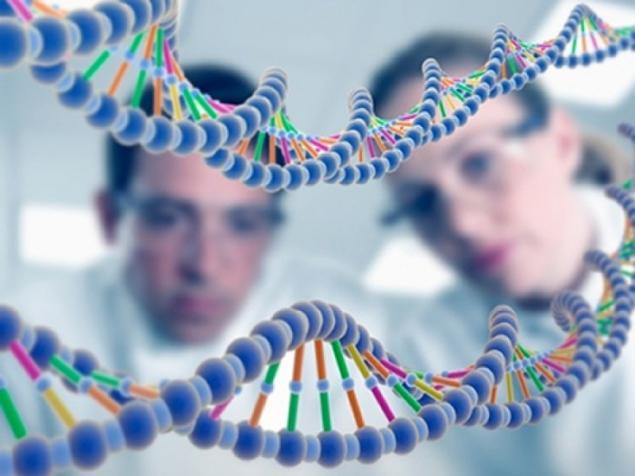
In the 19th century, phosphorus was commonly referred to as brain food, and from about 1970 onwards, its involvement in cellular regulation was at the heart of reductionist thinking. ATP, adenosine triphosphate, is considered as an energy source that provides cellular movement, and also provides energy to the “pumps” that support the state of life, as a source of cyclic adenosine monophosphate, which is a common activator of cells, as a donor of the phosphate group, which activates a large number of proteins in the “phosphorylation cascade”.When tissues are calcified in the process of aging, the responsibility for this is assigned to calcium (while ignoring the existence of calcium phosphate crystals in tissues) and therefore low-calcium diets are recommended. More recently, calcium has been blamed for the fact that calcium supplements did not give the expected effect, despite the fact that in addition to calcium, other substances such as citrate, phosphate, orotate, aspartate and lactate are also present in these supplements.
I have a different view of the “phosphorylation cascade” and other cellular functions of phosphate; it was formed from my understanding of the role of water in cellular physiology. A popular view is that the stimulus changes the shape of the receptor protein, which forces it to work as an enzyme, catalyzing the transition of the phosphate group from ATP to another protein, which in turn changes its shape and translates into an active state, resulting in the transfer of phosphate groups to other molecules or the removal of phosphates from active enzymes in chain reactions. This is a standard biochemistry that can be replicated in a test tube.
Since about 1970, when it was already well known about the involvement of phosphorylation in the activation of enzymes in the breakdown of glycogen, scientists began to notice that when muscle cells are reduced, the enzyme glycogen phosphorylase is instantly activated, and then phosphorylation occurs. The activation of the enzyme involves phosphorylation, so if something else first activated the enzyme (by changing its shape), then the addition of a phosphate group cannot be considered the sole cause of this process in the usual reductionist sense. Phosphorylation is one of the participants in a complex causal process. I saw this as a possible example of the effect that a changing water structure has on protein structure and function.
As you know, if the temperature drops below a certain value, enzymes suddenly lose activity. This is because soluble proteins take on a form in which their hydrophobic elements, i.e. fat-like side chains of amino acids, end up inside, and parts of the chain with water-soluble amino acids are placed outside, coming into contact with water. The “humidity” of water, its desire to reject the oily parts of the protein molecule decreases as the temperature drops, so some proteins have an unstable state in which the relatively hydrophobic group is no longer repelled by the colder water surrounding it.
In a living cell, water is always very close to the surface of fats or fat-like proteins. In a series of experiments that began in the 1960s, Walter Drost-Hansen showed that regardless of the nature of matter, water near the surface changes structurally, becoming less dense, more bulky. This water is more “lipophilic”, it adapts to the presence of fatty material, as if it is colder. Changes in the properties of water also affect the solubility of ions, with potassium solubility increasing and solubility of sodium, magnesium and calcium falling (Wiggins, 1973).
When a muscle contractes, it decreases in volume (Abbott and Baskin, 1962). Muscles also contract under extremely high pressure. In both cases, the productive reduction process involves a slight decrease in volume. During the contraction of a muscle or nerve, heat is released, which leads to an increase in temperature. During rest, heat is absorbed during the recovery process (Curtin and Woledge, 1974; Westphal, et al., 1999; Constable, et al. 1997). In the case of a nerve, following the heating caused by excitation, the nerve temperature drops below the initial temperature (Abbot, et al., 1965). Muscle stretching leads to energy absorption (Constable, et al., 1997). Such energetic changes, unaccompanied by chemical changes, have led some researchers to conclude that the creation of muscle tension is a process "driven by entropy" (Davis and Rodgers, 1995).
In 1858, Kelvin wrote about the physics of water in a soap bubble: "... if a film, such as a soap bubble, is stretched, ... it cools ..." This description also applies to the behavior of nerves and muscles that absorb energy at the time of relaxation (or lengthening) and release it when excited / contracted.
Several groups of experimenters over the past 60 years have tried to figure out what happens to the missing heat; some have suggested the presence of an electrical or osmotic storage, and some have shown that ATP is generated when stretched, and have therefore advocated the idea of chemical storage. The possibility of physical storage in the form of structural changes in the water-protein-fat system interacting with chemical changes such as ATP synthesis has not been studied.
In early studies of muscle chemistry and muscle contraction, it was found that adding ATP to a viscous solution of muscle extract reduced its viscosity, and muscle loss of ATP led to its hardening, as in rigor. If the pH value was not too acidic, then with a decrease in the content of ATP in the dead muscle, its reduction occurred. St. Györdy found that the muscle hardened by rigor became soft again when ATP was added.
Major rigor is extreme fatigue or energy depletion. In early studies, the phenomenon of “fatigue contracture” was described, in which the muscle reduced to the loss of the ability to respond to stimulation is reduced as much as possible (this condition is also called delayed relaxation). Ischemic contracture in the absence of circulation occurs when muscle glycogen is exhausted, and thus the anaerobic mechanism of ATP production stops (Kingsley, et al., 1991). Delayed hypothyroid muscle relaxation is another situation in which ATP is clearly required to relax. (In the Achilles tendon reflex test for hypothyroidism, the relaxation rate is slowed down, which can be seen visually.) T-wave delay on the electrocardiogram, diastolic contracture in heart failure is still the same process of delayed relaxation. By introducing the active thyroid hormone, T3, it is possible to quickly return to normal the relaxation rate, such a beneficial effect of the hormone is used in heart failure (Pingitore, et al., 2008; Wang, et al., 2006; Pantos, et al., 2007; Galli, et al., 2008).
Most of the magnesium in the cells is associated with ATP, the magnesium-ATP complex is a factor in muscle relaxation. Lack of ATP or magnesium leads to muscle spasms. When a cell is stimulated, ATP releases not only inorganic phosphate, but also magnesium. At a pH of more than 6.7, phosphate is twice ionized, exerting the same structural effect on water as magnesium, calcium and sodium: water molecules are strongly attracted to the concentrated electric charge of the ion. The increase in the concentration of free phosphate and magnesium counteracts the effect of fat and protein surfaces on the structure of water, leads to a decrease in the solubility of potassium in water, and also increases the “lipophobic” tendency of water to minimize contact with fats and fat-like areas on the surface of proteins, forcing proteins to rearrange.
Observations concerning the interactions in muscles and nerves of water, dissolved substances, and proteins offer a clear context for understanding contraction and conduction processes that is not found in familiar descriptions based on the concepts of cell membranes, pumps, and crossed bridges. I think these observations also offer a uniquely useful context for understanding the possible dangerous effects of excess phosphate in the body.
Some scientists (M. Thomson, J. Gunawardena, A. K. Manrai) show that the principle of acting masses helps to simplify the understanding of phosphorylation and dephosphorylation networks that are involved in cellular regulation. Regardless of protein phosphorylation, the presence of a phosphate ion in cellular water changes the ion selectivity of the cell, shifting the balance towards increased sodium and calcium absorption, reducing potassium and showing a tendency to depolarize and “activate” the cell.
Approximately 99% of publications that discuss muscle contraction do not address the role of water; water is also ignored when discussing energy production in mitochondria. Failure to produce mitochondrial energy leads to lipid peroxidation, activates inflammatory processes and can cause disintegration of the energy-producing structure. Increased concentration of phosphate reduces mitochondrial energy production (Duan and Karmazyn, 1989), causes lipid peroxidation (Kowaltowski, et al., 1996), triggers inflammatory processes, enhances tissue atrophy, fibrosis and cancer.
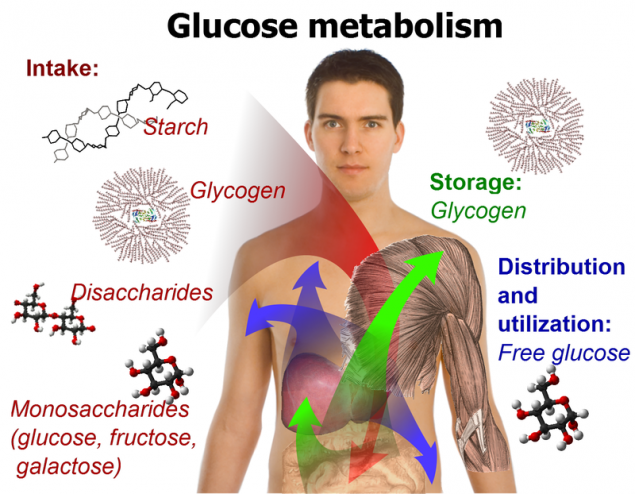
For twenty years, it has been known that a metabolic problem leading to loss of calcium in bones causes calcium buildup in soft tissues, such as blood vessels. Until recently, it was believed that phosphates play a passive role in the formation of calcium phosphate crystals, but some specific “mechanistic” effects have been identified.
For example, an increased concentration of phosphate leads to an increase in the inflammatory cytokine, osteopontin (Fatherazi, et al., 2009), which is known for its ability to activate the decalcification process in the bones and to participate in calcification processes in the arteries (Tousoulis, et al., 2012). Phosphates increase calcification in the kidneys (Bois and Selye, 1956), and osteopontin, due to the activation of inflammatory T cells, is involved in the development of glomerulonephritis and inflammatory skin reactions (Yu, et al., 1998).
The high content of phosphates in the diet increases the concentration of serum osteopontin, as well as serum phosphate and parathormone, contributes to the formation of skin tumors (Camalier, et al., 2010). In addition to activating cells and cellular systems, phosphates (like other ions with a high charge-to-size ratio, including citrate) are capable of activating viruses (Yamanaka, et al., 1995; Gouvea, et al., 2006). Aromatase, an enzyme that synthesizes estrogen, is sensitive to phosphate concentrations (Bellino and Holben, 1989).
Generally speaking, increased dietary phosphate enhances the activity of an important regulatory enzyme, protein kinase B, which promotes organ growth. A diet high in phosphate leads to an increase in liver (Xu et al., 2008) and lungs (Jin et al., 2007), supports the growth of lung cancer (Jin et al., 2009).But drastically restricting phosphates will not be the right solution, as their deficiency stimulates phosphate transport in cells, increasing cellular uptake of phosphates and having a similar effect as in conditions of excess phosphate, that is, increasing the growth of lung cancer (Xu et al., 2010).. The optimal content of phosphates in the diet and their share in the mineral composition of nutrition have not yet been determined.
An increase in phosphate levels slows down mitochondrial energy production, and a decrease in the concentration of phosphates in the intercellular space leads to an increase in the respiratory index and the effectiveness of ATP formation. To the same effect leads the "deficiency" of polyunsaturated fatty acids (Nogueira et al., 2001) and the consumption of fructose (Green et al., 1993; Lu et al., 1994).
In 1938, an experiment was conducted (Brown et al.) on a man who for six months received a diet of 2500 calories per day, consisting of sucrose syrup, a gallon of milk (partly in the form of grain cottage cheese), the juice of half an orange, as well as certain vitamins and minerals in the form of additives.
This experiment was supposed to demonstrate the irreplaceability of unsaturated fats. The members of the experimental group were very puzzled that surprisingly they did not observe normal fatigue at the end of the working day. He normalized high blood pressure and high cholesterol, and completely stopped migraines, which he suffered all his life. He had an increase in respiratory coefficient (carbon dioxide production increased) and resting metabolic rate. I think the most interesting result of this experiment is the reduction of phosphate in the blood of the subject. He had a fasting blood test twice. The serum neo-limited phosphorus content was 3.43 and 2.64 mg per 100 ml of plasma. At the end of the study, he returned to his normal diet, and his blood phosphorus levels were 4.2 mg/100 ml six months later. It can be assumed that the decrease in phosphates in the body occurred both due to a deficiency of “essential” unsaturated fatty acids, and due to the intake of high doses of sucrose.
In 2000, convinced of the dangerous properties of fructose, researchers suggested that switching to a diet with a magnesium deficiency would increase the harm caused by fructose. And eleven people took part in a six-month experiment, during which they received a diet high in corn starch or syrup, rich in fructose. They were also given other typical American foods, either extremely low in magnesium or with some deficiency. The conclusion reached by the researchers was clearly spelled out in the title of their paper and was that the combination described above significantly worsens the mineral balance in the body.
However, in the context of other studies on the effects of fructose on phosphates, I do not believe the above conclusions are correct. Even in the case of the extremely magnesium-poor diet, the balance of both magnesium and calcium was positive, from which it can be concluded that on average the subjects’ bodies consumed very little calcium and magnesium, people aged 22 to 40 do not seem to grow very much.
With a stable intake of both calcium and magnesium, with a significant excess of the absorbable level of calcium, it can be assumed that the minerals were absorbed in the bones. However, in the case of a “high-fructose” diet, their phosphate balance was slightly negative. If sugar had the same effect as in the William Brown experiment in 1938 (and in animal experiments), a slight decrease in phosphate could be explained by a decrease in its amount in the blood and other fluids, but as the experiment continued, this phenomenon must at some stage reflect changes in bone composition. When body fluids contain more carbon dioxide, calcium carbonate can be deposited in bones (Messier et al., 1979). Increased carbon dioxide levels can lead to a long-term negative phosphate balance due to the role that carbon dioxide plays in bone biochemistry when interacting with calcium and magnesium.
Another important property of carbon dioxide is its ability to regulate both calcium and phosphate simultaneously, increasing calcium absorption and retention (Canzanello et al., 1995) and enhancing phosphate release. Increased concentrations of carbon dioxide (as dissolved gas) and bicarbonate (sodium bicarbonate) lead to increased release of phosphates in the urine, even in the absence of parathyroid hormone. If the concentration of serum bicarbonate is below normal values, the reabsorption of phosphates by the kidneys increases significantly (Jehle et al., 1999). Acetazolamide increases the retention of carbon dioxide by the body, increasing the excretion of phosphates in the urine. A large proportion of calcium in the blood is a positively charged complex consisting of calcium and bicarbonate, its charge is equal to one (Hughes et al., 1984). Failure to handle this complex form of calcium leads to errors both in determining the level of calcium in the blood and in interpreting its physiological effects, including its action in the intercellular space. Hyperventilation can lead skeletal muscles into a convulsive state, constrict coves, excite platelets and other cells; removing carbon dioxide from the blood reduces the concentration of carbonic acid, altering the state and function of calcium. Hyperventilation leads to the growth of phosphate and parathyroid hormone, reduces the calcium content (Krapf et al., 1992).
Since estrogen tends to cause hyperventilation, and thus a decrease in carbon dioxide concentration, its role in phosphate metabolism should be studied more carefully. In the work of Han et al. (2002) and Xu et al. (2003), it was shown that estrogen increases the reabsorption of phosphate by the kidneys, but estrogen also leads to an increase in cortisol, which reduces reabsorption, so the effect of estrogen on the whole body should be taken into account.
The solubilizing effect on calcium from bicarbonate, combined with its ability to phosphaturia, may be responsible for the relaxing effect of carbon dioxide on blood vessels and smooth bronchial muscles, and also underlies the mechanism of preventing vascular calcification by thyroid hormone (Sato et al., 2005, Tatar, 2009, Kim et al., 2012). The elasticity of blood vessels and the heart is enhanced by carbon dioxide and, on the contrary, reduced with hypothyroidism, heart failure and under the influence of phosphate.
Fructose not only reduces the concentration of phosphate in the intercellular space, but also reduces the amount of phosphate absorbed in the gastrointestinal tract from food (Kirchner et al., 2008), and Milne-Nielsen studies conclude that it also increases the loss of phosphate through the kidneys. The “anti-aging” protein klotho enhances the ability of the kidneys to excrete phosphate and, like fructose, it supports energy production and thermogenesis (Mori et al., 2000).
A decrease in phosphate in the blood leads to a decrease in parathyroid hormone. Parathormone, by preventing the reabsorption of phosphate by the kidneys, leads to the production of serotonin by mast cells (and serotonin increases the renal reabsorption of phosphate) and possibly has other anti-inflammatory properties. For example, deletion of the PTH gene compensates for the negative effects (accelerated calcification and osteoporosis) of deletion of the klotho gene by preventing the growth of osteopontin (Yuan et al., 2012).
Niacinamide is another nutrient that reduces serum phosphate (Cheng et al., 2008), suppressing its absorption in the intestine (Katai et al., 1989) and reducing its reabsorption in the kidneys (Campbell et al., 1989). It is possible that the ability of niacinamide to reduce the level of free fatty acids by inhibiting lipolysis may play a role in the process of its effect on phosphate (similar to the phosphatoponizing effect in the deficiency of polyunsaturated fatty acids). Aspirin is another anti-lipolytic (de Zentella et al., 2002) that stimulates energy production from sugar and reduces phosphate, possibly contributing to magnesium retention (Yamada and Morohashi, 1986).
A diet containing enough calcium to limit the activity of the parathyroid glands and low amounts of phosphate and polyunsaturated fats, which uses sugar rather than starch as the main source of carbohydrates, as well as possible supplements of aspirin and niacinamide, should contribute to the fight against degenerative processes associated with high levels of phosphate: weakness, heart failure, discoordination of movements, hypogonadism, infertility, vascular calcification, emphysema, cancer, osteosis, osteosis, osteosis, 2010, drosis, and skin drosis (Oki, 2012, drosis, and derazka, and derazka, and droska, droska, and droscia, drosis, and drosis, 2012);The foods most rich in phosphate relative to their calcium content are muesli, legumes, meat and fish. Phosphate is added to many semi-finished products. Foods with a higher, safer ratio of calcium to phosphate are cabbage leaves and beets, many fruits, milk and cheese. Coffee, being a good source of magnesium, seems to be very effective in reducing phosphate due to its antagonism to adenosine. (Coulson et al., 1991).
Although elevated phosphate usually leads to vascular calcification (increases their stiffness and, accordingly, increases systolic blood pressure), in the case when most of the phosphate comes from food from milk and cheese, epidemiologically its effect is associated with a decrease in blood pressure (Takeda et al., 2012).
The toxicity of phosphate leads to some very interesting conclusions regarding stress and aging and helps explain the protective effects of carbon dioxide, thyroid hormone, sugar, niacinamide and calcium. Its toxicity suggests that the effects of other natural foods used as food additives should be carefully studied. For example, excess citric acid can activate dormant cancer cells (Havard et al., 2011) and lead to their malignancy (Blüml et al., 2011).Generally speaking, research to establish optimal ratios between minerals, fats, amino acids and other substances in foods, as well as studying their interaction with natural toxins, antinutrients and substances that destroy the body’s hormonal system, has barely begun.published
© Ray Pete.
P.S. And remember, just changing our consumption – together we change the world!
Source: //ekiri22.blogspot.com/2015/02/blog-post.html