81
How the nervous system works – scientists took a video
The activity of several hundred neurons in the worms and several thousand neurons in the fish’s brain was seen in real time.
To understand how the brain works, you need to accurately represent its structure. If we remember the peculiarities of the structure of nerve cells, their ability to form a great many intercellular contacts, it becomes clear that the structure of the brain here should be understood not only as a "large block" structure (cerebellum, thalamus, cortex, etc.), but also the entire system of connections between them. Of course, the brain has a large supply of plasticity: intercellular synapses in it appear and disappear. However, in the brain there are, so to speak, constant channels of communication that remain unchanged and which form the material basis of intracerebral information flows.
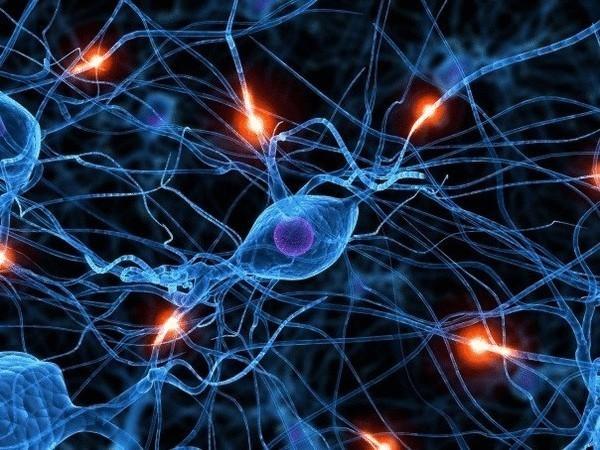
Until now, the only organism whose connections in the nervous system have been described in detail remains the nematode Caenorhabditis elegans. This worm has a nervous system of only 302 cells, so neuroscientists were able to figure out what it connects to quite quickly – by 1986, a complete map of the interneuronal connections of C. elegans was created. Of course, scientists soon thought about making a similar map for the human brain, but the human brain has not 302, but about 100 billion neurons, so you can imagine what a titanic task the researchers are facing. Moreover, neurons do not just form an amorphous network, they fold into functional zones that perform a particular task, and these zones, in turn, interact with each other at the macro level and obey some additional architectural rules. And all of this makes it incredibly difficult to map the brain.
Scientists are trying to solve this problem from different angles, sometimes quite unexpected methods. Last year, researchers at Stanford University managed to make the mouse brain and a fragment of the human brain almost transparent: nerve tissue was treated with a detergent so that only protein “skeletons” of nerve cells remained in it, and using fluorescent proteins, it was possible to trace the distribution of nerve processes from the outer layers of the cortex to the very interior of the brain.
On the other hand, the old method of mapping continues to be successful, where nerve tissue is sliced into thousands of layers each a few tens of micrometers thick, and then these layers are stared at, assessing the similarities and differences. After analyzing their structure, you can build a three-dimensional map of the brain with increased accuracy. Such work is carried out constantly, and brain atlases are gradually becoming more detailed. So, again last year, a team of scientists from the Jülich Research Center in Germany, together with colleagues from other research centers in Germany and Canada, managed to create a three-dimensional atlas of the human brain with a resolution of 20 micrometers – this map of the brain was 50 times more accurate than its predecessors.
Often, neuroscientists only deal with a single aspect of neural architecture, for example, trying to map the pathways between all areas of the brain. Here, too, managed to achieve significant success: just a month ago, researchers from the Allen Institute of the brain reported that they were able to determine the entire set of intracerebral connections, however, so far only for the mouse brain.
But let's say we know all the connections that exist in the brain, that we've created the most detailed three-dimensional atlas -- is that enough to understand how the brain works? Obviously not, because we won't know exactly how nerve impulses are distributed throughout the network of connections between neurons and large, small areas of the brain. As an analogy, computer chips can be used: we can look at them as much as we like, but from their appearance we cannot determine whether a computer is currently working with a video file or a text editor. To do this, we need to get inside the chip, “see” the electric currents that run along it. And in the same way, you have to go inside the neuron, you have to know the distribution of the impulse across the nerve cells to understand how it works.
Scientists have long been able to record and analyze the activity of nerve cells. As you can easily guess, observing the activity of a single neuron will tell us nothing – you need to know who it received the signal from and to whom it transmitted it, that is, the activity of the entire chain, or at least most of it. But neural circuits do not exist by themselves, they exchange information with each other. We can determine which group of neurons is involved, for example, in motor activity, but if we want to get a more complete picture, if we want to know how sensory input is converted into motor output, we need to look at the whole brain at once.
This is exactly what Robert Prevedel and his colleagues at the Institute of Molecular Pathology in Vienna and the Massachusetts Institute of Technology have tried to solve. And they managed to solve it, however, not yet on the human brain, and not on the mouse, but on the same simple nervous system of the nematode C. elegans and on the developing brain of fry zebrafish.
The researchers modified the worms and fish so that their neurons synthesized a fluorescent protein: this protein glowed when changes in the level of calcium ions inside the cell. As is known, during the excitation and propagation of an electric impulse, ions redistribute on both sides of the neural membrane - in fact, changes in the concentration of ions are the basis of the nerve impulse. You can monitor the work of the neuron by the movement of ions in and out of the cell, and if we have a special luminous protein that is sensitive to such redistributions of ions, then you can generally monitor the work of the nerve cell with your own eyes, albeit with a microscope.
In fact, this technology has long been used to study nerve impulses, but until now it has been used on a small number of nerve cells. This time, the task of the scientists was to use a glowing protein to monitor the work of the entire nervous system at once, so that the image was three-dimensional, and that the activity of the nervous system could be recorded at high speed. This was done using a special microscopic method that allows you to take 50 pictures per second, which were then mounted in a 3-D image. You can see which neurons in the worm are at rest, which are when the worm is crawling, and which are when it feels some kind of smell or tactile stimulation. (A video of the creeping glowing nematode can be viewed on YouTube.
In the nematode C. elegans, the entire nervous system, from head to tail, has only 302 neurons. The fry of zebrafish already have 100,000 nerve cells, and scientists could not take into account the activity of all at once, limiting themselves so far to only five thousand (which is still not bad compared to three hundred neurons in worms).
The researchers note the weak point of the method they developed: it allows you to see some average activity of the entire nerve cell, but it no longer allows you to see the activity of a single neural process, axon or dendrite. However, the researchers hope that they will be able to improve the technology and further detail the image.
This work is essentially methodical (and it was published in Nature Methods), but with the help of such a method it will be possible to learn a lot about the functioning of neural networks on the scale of, if not the entire brain, then at least part of it. Of course, we can say that the nervous system of nematodes and zebrafish is immeasurably simpler than that of humans, but, firstly, this method can be applied to the brain of some experimental mammals, and, secondly, some patterns of the nervous system can be investigated at the level of simple worms. Once again, we point out the main feature of this approach: we record the work of the entire nervous system (or at least a fairly large proportion of neurons) in real time. And thanks to the fact that the activity of nerve cells is visible here without time delays, we can more accurately imagine the information processes that occur in the nervous system.
However, this dilemma between the number of neurons and their activity is still not fully solved: either we see a lot of neurons, but we can not assess their work in detail, or vice versa – we see all the details of the transmission of impulses, but only between a limited number of nerve cells. We can only hope for further scientific and technological progress.
Source: nkj.ru
Microsoft decided to join the "smart" home
Chinese "rice theory": as a native speaker form the national mentality