474
10 unusual States of matter
Most people can easily call the three classic States of matter: solid, liquid and gaseous. Those who know a little science, add to this three more plasma.
But over time, scientists have expanded the list of possible States of matter beyond those four. In the process we learned a lot about the Big Bang, lightsaber and secret state of matter, latent in the humble chicken.
Amorphous and solid
Amorphous solids is quite an interesting subgroup of well known solid state. In a normal solid object, the molecules are well organized and not really have space to move. It gives a solid substance with a high viscosity, which is a measure of resistance to flow. Liquids, on the other hand, are disorganized molecular structure that allows them to run, to flow, change shape and take the form of the vessel in which they are located. Amorphous solids are somewhere between these two States. In the process of vitrification the liquid cools and the viscosity increases until the moment when the substance is not flowing like a liquid, but its molecules remain disordered and do not adopt a crystalline structure like ordinary solids.
The most common example of amorphous solid is glass. For thousands of years people have been making glass from silica. When the silica glass is cooled from the liquid state, it is actually not solidified, when it descends below the melting point. When the temperature drops, viscosity increases, the substance seems to be harder. However, its molecules are still disordered. And then the glass becomes amorphous and solid at the same time. This transition has allowed artisans to create beautiful and surreal glass structure.
What is the functional difference between amorphous solids and regular solid? In everyday life, it is not particularly noticeable. Glass seems completely solid until you examine it on a molecular level. And the myth that glass flows over time, not worth a penny. Most often this myth is reinforced by the arguments that the old glass in churches seems to be thicker at the bottom, but this is due to the imperfection of the glassblowing process at the time of the creation of these glasses. However, to study amorphous solids like glass is interesting from a scientific point of view for the study of phase transitions and molecular structure.
Supercritical fluids (fluids)
Most of the phase transitions occurs at a certain temperature and pressure. It is well known that increasing temperature eventually turns a liquid into a gas. However, when the pressure increases with temperature, the liquid jumps into the realm of supercritical fluids that have properties of both gas and liquid. For example, supercritical fluid can pass through solids like a gas, but can also act as a solvent, as a liquid. Interestingly, the supercritical liquid can be made more similar to a gas or liquid, depending on the combination of pressure and temperature. This allowed the scientists to find many uses for supercritical fluids.
Although supercritical fluids are not as common as amorphous solids, you probably interact with them as often as with glass. Supercritical carbon dioxide is like the brewing company for its ability to act as a solvent in the interaction with the hops, and coffee companies use it to produce the best coffee without the caffeine. Supercritical fluids are also used for more efficient hydrolysis to power plants and worked at higher temperatures. In General, you are probably using the by-products of supercritical fluids every day.
A degenerate gas
Although amorphous solids at least meet on the planet Earth, degenerate matter is found only in certain types of stars. A degenerate gas exists when the external pressure of the substance is determined not by the temperature, as on Earth, and complex quantum principles, in particular the Pauli principle. Because of this external pressure to degeneracy will persist, even if the temperature drops to absolute zero. There are two main types of degeneracy: electron degenerate and neutron-degenerate matter.
Electron-degenerate matter exists mostly in white dwarfs. It is formed in the core of the star when the mass of the matter around the core tries to shrink the core electrons to the lowest energy state. However, in accordance with the Pauli principle, two identical particles cannot be in the same energy state. Thus, the particles are "pushing" stuff around the nucleus, creating pressure. This is only possible if the mass of the star is less than 1.44 times the mass of the Sun. When a star exceeds this limit (known as the Chandrasekhar limit), it just collapses into a neutron star or a black hole.
When a star collapses and becomes a neutron star, it is no more electron degeneracy, it is composed of neutron-degenerate matter. Since a neutron star is heavy, the electrons merge with the protons in its nucleus, forming neutrons. Free neutrons (neutrons not bound in the atomic nucleus) have a half-life of 10.3 minutes. But at the core of a neutron star the mass of the star allows the neutrons exist outside the nuclei, forming a neutron-degenerate matter.
Other exotic forms of degenerate matter also exist, including strange matter that may exist in rare form stars — quark stars. Quark star — a stage between a neutron star and a black hole, where the quarks in the nucleus untied and form a soup of free quarks. We have not yet observed this type of star, but physicists admit their existence.
Superfluidity
Get back to Earth to discuss the superfluid liquid. Superfluidity is a state of matter that exists in certain isotopes of helium, lithium and rubidium cooled to almost absolute zero. This state is similar to the condensate the Bose — Einstein (Bose-Einstein condensate, BEC), with a few differences. Some BEC superfluid, and the superfluid state, some are BACK but not all of them are identical.
Liquid helium is known for its superfluidity. When helium is cooled to point "lambda" -270 degrees Celsius, the liquid becomes superfluid. If cooling a large part of the substances to a certain point, the attraction between the atoms exceeds the thermal vibration in the substance, allowing them to form a solid structure. But the helium atoms interact so weakly that they can remain liquid at a temperature of almost absolute zero. So, at this temperature, the characteristics of the individual atoms overlap, giving rise to strange properties of superfluidity.
Superfluid substances have no internal viscosity. The superfluid of a substance placed in a test tube, begin to creep up the sides of the tube, seemingly breaking the laws of gravity and surface tension. Liquid helium easily leaks because it can slip even through microscopic holes. Superfluidity also possesses a strange thermodynamic properties. In this state of matter have a zero thermodynamic entropy and infinite thermal conductivity. This means that the two superfluid substance cannot be thermally different. If you add in the super-fluid substance of heat, it will hold it so fast that are formed of a heat wave, not typical for ordinary liquids.
Condensate Bose — Einstein
The condensate of Bose — Einstein is probably one of the most famous strange forms of matter. First, we need to understand what bosons and fermions. A fermion is a particle with half-integer spin (e.g. electron) or composite particle (like a proton). These particles obey the Pauli principle, which allow for the existence of electron-degenerate matter. Boson, however, has a full integer spin, and one quantum state can span multiple bosons. Bosons include any particles carry forces (like photons), as well as some atoms, including helium-4 and other gases. Items in this category are known as bosonic atoms.
In the 1920-ies albert Einstein took as the basis of the work of the Indian physics Satyendra Nath Bose to offer a new form of matter. The original Einstein's theory lay in the fact that if you cool certain elementary gases to a temperature in a fraction of a degree above absolute zero, their wave functions will merge, creating one "swashata". The substance will exhibit quantum effects on a macroscopic level. But only in the 1990-ies appeared the technology needed for the cooling elements to such temperatures. In 1995, scientists Eric Cornell and Carl wieman were able to combine 2000 of atoms in the condensate Bose — Einstein, which was large enough to be seen with a microscope.
Condensates Bose-Einstein closely related to the superfluid substances, but also have their own a set of unique properties. It's funny that BACK can slow down the usual speed of light. In 1998, Harvard scientist Lene Hau was able to slow light to 60 kilometers per hour, passing the laser through a sample of the cigar-shaped BEC. In later experiments the group How managed to completely stop light in the BACK, turning off the laser when the light passed through the sample. These experiments opened a new field of communications based on light and quantum computing.
Metals Jahn — Teller
Metals Jahn — teller is the latest child in a world of States of matter, as scientists were able to successfully create them for the first time only in 2015. If the experiments are confirmed by other laboratories, these metals can change the world, as they have properties as insulator and superconductor.
Scientists led by chemist Kosmas Prassides experimented by introducing the rubidium in the structure of the molecules of carbon-60 (in the common people known as fullerenes) that have led to the fact that fullerenes are taking a new form. This metal is named in honor of the Jahn — teller effect, which describes how pressure can change the geometric shape of the molecules in the new electronic configurations. In chemistry, pressure is not only due to the compression of something, but by adding new atoms or molecules in the pre-existing structure, altering its basic properties.
When the research group of Prassides started adding the rubidium molecule of carbon-60, carbon molecules changed from insulators to semiconductors. However, because of the effect of Jahn — teller molecules was trying to stay in the old configuration, which created a substance that was trying to be the infirmary, but had the electrical properties of the superconductor. The transition between the insulator and the superconductor are never considered until I started these experiments.
Interested in metals Jahn — teller the fact that they become superconductors at higher temperatures (-135 degrees Celsius, not when 243,2 degrees, as usual). It brings them to the acceptable levels for mass production and experimentation. If it checks out, maybe we'll be one step closer to creating superconductors that operate at room temperature, which in turn will revolutionize many sectors of our life.
Photonic substance
For many decades it was believed that photons are massless particles that do not interact. Nevertheless, over the past few years, scientists at MIT and Harvard have discovered new ways to "endow" the light weight and even create "molecules of light", which bounce off each other and connect together. Some felt that this is the first step towards the creation of the lightsaber.
Science of photon-matter a little more difficult, but to comprehend it. Scientists began to create photonic matter of experimenting with supercooled rubidium gas. When the photon sweep gas, it is reflected and interacts with the rubidium molecules, losing energy and slowing down. In the end, the photon leaves the cloud is very slow.
Strange things begin to happen when you miss two photons through the gas that gives rise to the phenomenon known as Rydberg blockade. When the atom is excited by a photon, nearby atoms cannot be excited to the same degree. An excited atom is in the path of the photon. To a nearby atom could be excited by a second photon, the first photon must pass through the gas. Photons normally do not interact with each other, but meeting with Rydberg blockade, they push each other through the gas, exchanging energy and interacting with each other. From the outside it seems that photons have mass and they act as a single molecule, but are in fact massless. When the photons out of gas, they seem to be mingled, like the molecule of light.
The practical application of photonic matter is still in question, but it will certainly be found. Maybe even a lightsaber.
Unordered sverhdorogoy
Trying to determine if the substance is in new condition, scientists look at the structure of matter and its properties. In 2003, Salvatore Torquato and Frank Stillinger of Princeton University have proposed a new state of matter known as disordered sverhdorogoy. Although this phrase seems an oxymoron, at its core it involves a new type of substance that seems disordered, upon closer examination, but sverhodarennym and structured from afar. The substance should have the properties of a crystal and liquid. At first glance, this is already in plasmas and liquid hydrogen, but recently scientists have discovered a natural example where nobody expected it: in the chicken eye.
In chickens there are five cones in the retina. Find four color and one responsible for the levels of light. However, unlike the human eye, or a hexagonal eyes of insects, these cones are scattered randomly, have no real order. This happens because the cones in a chicken's eye have the exclusion zone around, and they do not allow the two cones of the same type to be around. Because of the exclusion zone and the shape of the cones they are unable to form an ordered crystalline structure (in solids), but when all cones are considered as a whole, it appears that they have a highly ordered pattern, as seen in the images below Princeton. Thus, we can describe these cones in the retina of chicken eyes like liquid upon closer examination and as solid matter when viewed from afar. This differs from amorphous solids, which we have discussed above, sverhdorogie since this material will act as a liquid, and amorphous solid — no.
Scientists are still exploring this new state of matter, as it, among other things, may be more prevalent than was believed originally. Now scientists at Princeton University are trying to adapt sverhdorogie such materials to create self-organizing structures and detectors of light that react to light with a certain wavelength.
String network
What state of matter is the vacuum of space? Most people don't think about it but in the last ten years Xiao gang-Wen from the Massachusetts Institute of technology and Michael Levin of Harvard proposed a new state of matter, which could lead us to the discovery of fundamental particles after electrons.
The way to develop the model, a string-net liquid began in the mid-90's, when a group of scientists proposed the so-called quasiparticles, which seemed to have appeared in the experiment, when the electrons passed between the two semiconductors. Arose a stir, because the quasi-particles acted as if they had a fractional charge that seemed impossible for physics at the time. The researchers analyzed the data and suggested that the electron is a fundamental particle of the Universe and that there are fundamental particles, which we have not yet discovered. This work brought him the Nobel prize, but it later emerged that the results of their work crept error in the experiment. On the quasiparticles forgot.
But not all. Wen and Levin took up the idea of quasi-particles and proposed a new state of matter, the string network. The main feature of this state is quantum entanglement. As in the case of disordered sverhdorogoy, if you close look at the string-net matter, it is similar to an unordered set of electrons. But if you look at it as a whole structure, you will see high ordering due to quantum-entangled properties of electrons. Wen and Levin then expanded its work to cover other particles and properties of entanglement.
After a computer model for a new state of matter, Wen and Levin found that the ends of the string-nets can produce a variety of subatomic particles, including the legendary "quasiparticles". An even bigger surprise was the fact that during vibration of the string-network substance it does so in accordance with Maxwell's equations, is responsible for the light. Wen and Levin suggested that the space is filled with the string networks of entangled subatomic particles, and that the ends of the string-networks represent the subatomic particles that we observe. They also suggested that a string-net liquid can ensure the existence of light. If the vacuum of space is filled with a string-net liquid, it can allow us to unite light and matter.
All this may seem very far-fetched, but in 1972 (decades before string-network proposals) geologists discovered in Chile a strange material — herbertsmithite. In this mineral the electrons form a triangular structure, which seems to contradict everything we know about the interaction of electrons with each other. In addition, this triangular structure was predicted in the framework of string-net models, and scientists working with artificial herbertsmithite to accurately confirm the model.
The quark-gluon plasma
Speaking about the latest status of the substances in this list, consider the state from which it all began: the quark-gluon plasma. In the early Universe the state of matter significantly differed from the classical. First, a little history.
A quark is an elementary particle that we find inside hadrons (e.g. protons and neutrons). Hadrons consist of either three quarks or one quark and one antiquark. Quarks have fractional charges and are held together by gluons, which are the exchange particles of the strong nuclear interaction.
We do not see free quarks in nature, but immediately after the Big Bang within fractions of free quarks and gluons existed. During this time the temperature of the Universe was so high that quarks and gluons were moving almost at the speed of light. During this period, the universe was entirely from this hot quark-gluon plasma. After another fraction of a second the universe had cooled enough to form heavy particles like hadrons, and quarks began to interact, and gluons. From this moment began the formation of the known Universe, the hadrons began to contact with electrons, creating a primitive atoms.
In the modern Universe, scientists tried to recreate the quark-gluon plasma in large particle accelerators. In the course of these experiments heavy particles like hadrons collided with each other, creating the temperature at which quarks were separated for a short time. In the course of these experiments, we learned a lot about the properties of the quark-gluon plasma, in which absolutely no friction and was more like a liquid than a regular plasma. Experiments with an exotic state of matter allows us to learn a lot about how and why our universe was formed as we know it.published
P. S. And remember, just changing your mind — together we change the world! ©
Source: hi-news.ru
But over time, scientists have expanded the list of possible States of matter beyond those four. In the process we learned a lot about the Big Bang, lightsaber and secret state of matter, latent in the humble chicken.
Amorphous and solid
Amorphous solids is quite an interesting subgroup of well known solid state. In a normal solid object, the molecules are well organized and not really have space to move. It gives a solid substance with a high viscosity, which is a measure of resistance to flow. Liquids, on the other hand, are disorganized molecular structure that allows them to run, to flow, change shape and take the form of the vessel in which they are located. Amorphous solids are somewhere between these two States. In the process of vitrification the liquid cools and the viscosity increases until the moment when the substance is not flowing like a liquid, but its molecules remain disordered and do not adopt a crystalline structure like ordinary solids.
The most common example of amorphous solid is glass. For thousands of years people have been making glass from silica. When the silica glass is cooled from the liquid state, it is actually not solidified, when it descends below the melting point. When the temperature drops, viscosity increases, the substance seems to be harder. However, its molecules are still disordered. And then the glass becomes amorphous and solid at the same time. This transition has allowed artisans to create beautiful and surreal glass structure.
What is the functional difference between amorphous solids and regular solid? In everyday life, it is not particularly noticeable. Glass seems completely solid until you examine it on a molecular level. And the myth that glass flows over time, not worth a penny. Most often this myth is reinforced by the arguments that the old glass in churches seems to be thicker at the bottom, but this is due to the imperfection of the glassblowing process at the time of the creation of these glasses. However, to study amorphous solids like glass is interesting from a scientific point of view for the study of phase transitions and molecular structure.
Supercritical fluids (fluids)
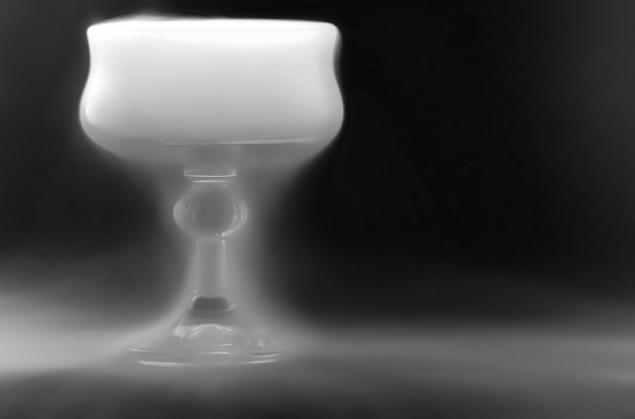
Most of the phase transitions occurs at a certain temperature and pressure. It is well known that increasing temperature eventually turns a liquid into a gas. However, when the pressure increases with temperature, the liquid jumps into the realm of supercritical fluids that have properties of both gas and liquid. For example, supercritical fluid can pass through solids like a gas, but can also act as a solvent, as a liquid. Interestingly, the supercritical liquid can be made more similar to a gas or liquid, depending on the combination of pressure and temperature. This allowed the scientists to find many uses for supercritical fluids.
Although supercritical fluids are not as common as amorphous solids, you probably interact with them as often as with glass. Supercritical carbon dioxide is like the brewing company for its ability to act as a solvent in the interaction with the hops, and coffee companies use it to produce the best coffee without the caffeine. Supercritical fluids are also used for more efficient hydrolysis to power plants and worked at higher temperatures. In General, you are probably using the by-products of supercritical fluids every day.
A degenerate gas
Although amorphous solids at least meet on the planet Earth, degenerate matter is found only in certain types of stars. A degenerate gas exists when the external pressure of the substance is determined not by the temperature, as on Earth, and complex quantum principles, in particular the Pauli principle. Because of this external pressure to degeneracy will persist, even if the temperature drops to absolute zero. There are two main types of degeneracy: electron degenerate and neutron-degenerate matter.
Electron-degenerate matter exists mostly in white dwarfs. It is formed in the core of the star when the mass of the matter around the core tries to shrink the core electrons to the lowest energy state. However, in accordance with the Pauli principle, two identical particles cannot be in the same energy state. Thus, the particles are "pushing" stuff around the nucleus, creating pressure. This is only possible if the mass of the star is less than 1.44 times the mass of the Sun. When a star exceeds this limit (known as the Chandrasekhar limit), it just collapses into a neutron star or a black hole.
When a star collapses and becomes a neutron star, it is no more electron degeneracy, it is composed of neutron-degenerate matter. Since a neutron star is heavy, the electrons merge with the protons in its nucleus, forming neutrons. Free neutrons (neutrons not bound in the atomic nucleus) have a half-life of 10.3 minutes. But at the core of a neutron star the mass of the star allows the neutrons exist outside the nuclei, forming a neutron-degenerate matter.
Other exotic forms of degenerate matter also exist, including strange matter that may exist in rare form stars — quark stars. Quark star — a stage between a neutron star and a black hole, where the quarks in the nucleus untied and form a soup of free quarks. We have not yet observed this type of star, but physicists admit their existence.
Superfluidity
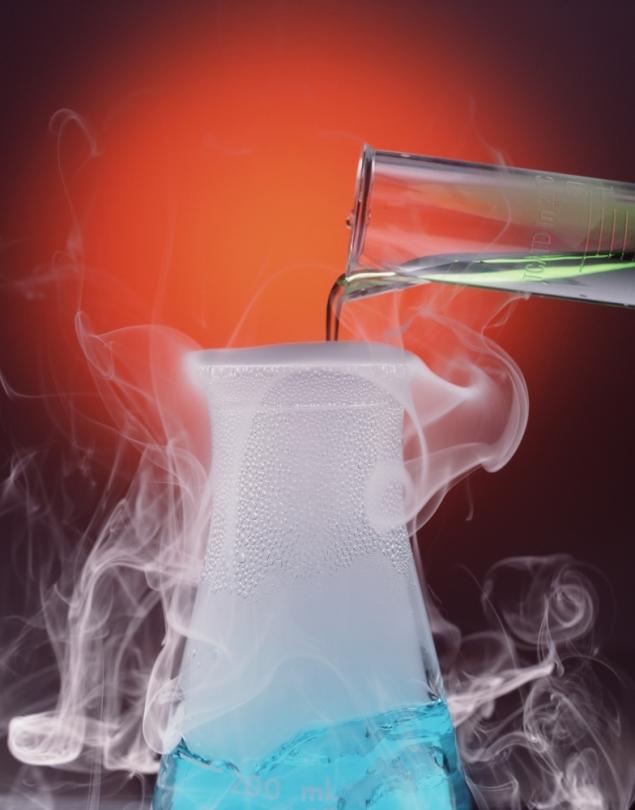
Get back to Earth to discuss the superfluid liquid. Superfluidity is a state of matter that exists in certain isotopes of helium, lithium and rubidium cooled to almost absolute zero. This state is similar to the condensate the Bose — Einstein (Bose-Einstein condensate, BEC), with a few differences. Some BEC superfluid, and the superfluid state, some are BACK but not all of them are identical.
Liquid helium is known for its superfluidity. When helium is cooled to point "lambda" -270 degrees Celsius, the liquid becomes superfluid. If cooling a large part of the substances to a certain point, the attraction between the atoms exceeds the thermal vibration in the substance, allowing them to form a solid structure. But the helium atoms interact so weakly that they can remain liquid at a temperature of almost absolute zero. So, at this temperature, the characteristics of the individual atoms overlap, giving rise to strange properties of superfluidity.
Superfluid substances have no internal viscosity. The superfluid of a substance placed in a test tube, begin to creep up the sides of the tube, seemingly breaking the laws of gravity and surface tension. Liquid helium easily leaks because it can slip even through microscopic holes. Superfluidity also possesses a strange thermodynamic properties. In this state of matter have a zero thermodynamic entropy and infinite thermal conductivity. This means that the two superfluid substance cannot be thermally different. If you add in the super-fluid substance of heat, it will hold it so fast that are formed of a heat wave, not typical for ordinary liquids.
Condensate Bose — Einstein
The condensate of Bose — Einstein is probably one of the most famous strange forms of matter. First, we need to understand what bosons and fermions. A fermion is a particle with half-integer spin (e.g. electron) or composite particle (like a proton). These particles obey the Pauli principle, which allow for the existence of electron-degenerate matter. Boson, however, has a full integer spin, and one quantum state can span multiple bosons. Bosons include any particles carry forces (like photons), as well as some atoms, including helium-4 and other gases. Items in this category are known as bosonic atoms.
In the 1920-ies albert Einstein took as the basis of the work of the Indian physics Satyendra Nath Bose to offer a new form of matter. The original Einstein's theory lay in the fact that if you cool certain elementary gases to a temperature in a fraction of a degree above absolute zero, their wave functions will merge, creating one "swashata". The substance will exhibit quantum effects on a macroscopic level. But only in the 1990-ies appeared the technology needed for the cooling elements to such temperatures. In 1995, scientists Eric Cornell and Carl wieman were able to combine 2000 of atoms in the condensate Bose — Einstein, which was large enough to be seen with a microscope.
Condensates Bose-Einstein closely related to the superfluid substances, but also have their own a set of unique properties. It's funny that BACK can slow down the usual speed of light. In 1998, Harvard scientist Lene Hau was able to slow light to 60 kilometers per hour, passing the laser through a sample of the cigar-shaped BEC. In later experiments the group How managed to completely stop light in the BACK, turning off the laser when the light passed through the sample. These experiments opened a new field of communications based on light and quantum computing.
Metals Jahn — Teller
Metals Jahn — teller is the latest child in a world of States of matter, as scientists were able to successfully create them for the first time only in 2015. If the experiments are confirmed by other laboratories, these metals can change the world, as they have properties as insulator and superconductor.
Scientists led by chemist Kosmas Prassides experimented by introducing the rubidium in the structure of the molecules of carbon-60 (in the common people known as fullerenes) that have led to the fact that fullerenes are taking a new form. This metal is named in honor of the Jahn — teller effect, which describes how pressure can change the geometric shape of the molecules in the new electronic configurations. In chemistry, pressure is not only due to the compression of something, but by adding new atoms or molecules in the pre-existing structure, altering its basic properties.
When the research group of Prassides started adding the rubidium molecule of carbon-60, carbon molecules changed from insulators to semiconductors. However, because of the effect of Jahn — teller molecules was trying to stay in the old configuration, which created a substance that was trying to be the infirmary, but had the electrical properties of the superconductor. The transition between the insulator and the superconductor are never considered until I started these experiments.
Interested in metals Jahn — teller the fact that they become superconductors at higher temperatures (-135 degrees Celsius, not when 243,2 degrees, as usual). It brings them to the acceptable levels for mass production and experimentation. If it checks out, maybe we'll be one step closer to creating superconductors that operate at room temperature, which in turn will revolutionize many sectors of our life.
Photonic substance
For many decades it was believed that photons are massless particles that do not interact. Nevertheless, over the past few years, scientists at MIT and Harvard have discovered new ways to "endow" the light weight and even create "molecules of light", which bounce off each other and connect together. Some felt that this is the first step towards the creation of the lightsaber.
Science of photon-matter a little more difficult, but to comprehend it. Scientists began to create photonic matter of experimenting with supercooled rubidium gas. When the photon sweep gas, it is reflected and interacts with the rubidium molecules, losing energy and slowing down. In the end, the photon leaves the cloud is very slow.
Strange things begin to happen when you miss two photons through the gas that gives rise to the phenomenon known as Rydberg blockade. When the atom is excited by a photon, nearby atoms cannot be excited to the same degree. An excited atom is in the path of the photon. To a nearby atom could be excited by a second photon, the first photon must pass through the gas. Photons normally do not interact with each other, but meeting with Rydberg blockade, they push each other through the gas, exchanging energy and interacting with each other. From the outside it seems that photons have mass and they act as a single molecule, but are in fact massless. When the photons out of gas, they seem to be mingled, like the molecule of light.
The practical application of photonic matter is still in question, but it will certainly be found. Maybe even a lightsaber.
Unordered sverhdorogoy
Trying to determine if the substance is in new condition, scientists look at the structure of matter and its properties. In 2003, Salvatore Torquato and Frank Stillinger of Princeton University have proposed a new state of matter known as disordered sverhdorogoy. Although this phrase seems an oxymoron, at its core it involves a new type of substance that seems disordered, upon closer examination, but sverhodarennym and structured from afar. The substance should have the properties of a crystal and liquid. At first glance, this is already in plasmas and liquid hydrogen, but recently scientists have discovered a natural example where nobody expected it: in the chicken eye.
In chickens there are five cones in the retina. Find four color and one responsible for the levels of light. However, unlike the human eye, or a hexagonal eyes of insects, these cones are scattered randomly, have no real order. This happens because the cones in a chicken's eye have the exclusion zone around, and they do not allow the two cones of the same type to be around. Because of the exclusion zone and the shape of the cones they are unable to form an ordered crystalline structure (in solids), but when all cones are considered as a whole, it appears that they have a highly ordered pattern, as seen in the images below Princeton. Thus, we can describe these cones in the retina of chicken eyes like liquid upon closer examination and as solid matter when viewed from afar. This differs from amorphous solids, which we have discussed above, sverhdorogie since this material will act as a liquid, and amorphous solid — no.
Scientists are still exploring this new state of matter, as it, among other things, may be more prevalent than was believed originally. Now scientists at Princeton University are trying to adapt sverhdorogie such materials to create self-organizing structures and detectors of light that react to light with a certain wavelength.
String network
What state of matter is the vacuum of space? Most people don't think about it but in the last ten years Xiao gang-Wen from the Massachusetts Institute of technology and Michael Levin of Harvard proposed a new state of matter, which could lead us to the discovery of fundamental particles after electrons.
The way to develop the model, a string-net liquid began in the mid-90's, when a group of scientists proposed the so-called quasiparticles, which seemed to have appeared in the experiment, when the electrons passed between the two semiconductors. Arose a stir, because the quasi-particles acted as if they had a fractional charge that seemed impossible for physics at the time. The researchers analyzed the data and suggested that the electron is a fundamental particle of the Universe and that there are fundamental particles, which we have not yet discovered. This work brought him the Nobel prize, but it later emerged that the results of their work crept error in the experiment. On the quasiparticles forgot.
But not all. Wen and Levin took up the idea of quasi-particles and proposed a new state of matter, the string network. The main feature of this state is quantum entanglement. As in the case of disordered sverhdorogoy, if you close look at the string-net matter, it is similar to an unordered set of electrons. But if you look at it as a whole structure, you will see high ordering due to quantum-entangled properties of electrons. Wen and Levin then expanded its work to cover other particles and properties of entanglement.
After a computer model for a new state of matter, Wen and Levin found that the ends of the string-nets can produce a variety of subatomic particles, including the legendary "quasiparticles". An even bigger surprise was the fact that during vibration of the string-network substance it does so in accordance with Maxwell's equations, is responsible for the light. Wen and Levin suggested that the space is filled with the string networks of entangled subatomic particles, and that the ends of the string-networks represent the subatomic particles that we observe. They also suggested that a string-net liquid can ensure the existence of light. If the vacuum of space is filled with a string-net liquid, it can allow us to unite light and matter.
All this may seem very far-fetched, but in 1972 (decades before string-network proposals) geologists discovered in Chile a strange material — herbertsmithite. In this mineral the electrons form a triangular structure, which seems to contradict everything we know about the interaction of electrons with each other. In addition, this triangular structure was predicted in the framework of string-net models, and scientists working with artificial herbertsmithite to accurately confirm the model.
The quark-gluon plasma
Speaking about the latest status of the substances in this list, consider the state from which it all began: the quark-gluon plasma. In the early Universe the state of matter significantly differed from the classical. First, a little history.
A quark is an elementary particle that we find inside hadrons (e.g. protons and neutrons). Hadrons consist of either three quarks or one quark and one antiquark. Quarks have fractional charges and are held together by gluons, which are the exchange particles of the strong nuclear interaction.
We do not see free quarks in nature, but immediately after the Big Bang within fractions of free quarks and gluons existed. During this time the temperature of the Universe was so high that quarks and gluons were moving almost at the speed of light. During this period, the universe was entirely from this hot quark-gluon plasma. After another fraction of a second the universe had cooled enough to form heavy particles like hadrons, and quarks began to interact, and gluons. From this moment began the formation of the known Universe, the hadrons began to contact with electrons, creating a primitive atoms.
In the modern Universe, scientists tried to recreate the quark-gluon plasma in large particle accelerators. In the course of these experiments heavy particles like hadrons collided with each other, creating the temperature at which quarks were separated for a short time. In the course of these experiments, we learned a lot about the properties of the quark-gluon plasma, in which absolutely no friction and was more like a liquid than a regular plasma. Experiments with an exotic state of matter allows us to learn a lot about how and why our universe was formed as we know it.published
P. S. And remember, just changing your mind — together we change the world! ©
Source: hi-news.ru