541
Electric highway of the future
In 2003, the EU has been much Desertec representing then a vision of the translation of Europe on the rails of renewable energy. The basis of “green energy” the EU was supposed to be a thermal power plant with a concentration of solar energy, located in the Sahara desert, able to store energy at least to the evening consumption peak, when the normal photovoltaics does not work anymore. Feature of the project was to be a powerful transmission line (PTL) for tens of gigawatts, with a range from 2 to 5 thousand km.
SES of this kind were to become the main European renewable energy industry.
The project lasted about 10 years, and was then abandoned by the companies-founders, as reality European green energy was completely different and more prosaic — Chinese photovoltaics and ground vetrogeneratory placed in Europe itself, and the idea is to pull the energy of the line through Libya and Syria — are too optimistic.
Planned in the framework of the desertec transmission lines: three main areas with a capacity of 3x10 gigawatts (in the picture one of the weaker versions 3x5) and several submarine cables.
However, powerful lines appeared in the "desertec" is no accident (funny, incidentally, that the area of land under power lines in the project was more land under the SES) is one of the key technologies that could enable renewable energy generation to grow to an overwhelming proportion, and Vice versa: in the absence of the technology of transmission of energy over long distances RES, quite possibly, doomed to no more than a fraction of 30-40% in the energy sector of Europe.
Mutual synergy transcontinental transmission lines and renewable energy is quite well visible in the models (for example, a giant model of the LUT, and also model Vyacheslav Laktyushina): the Union of many areas of wind generation, disposed in 1-2-3 thousands of kilometers from each other, destroys the cross-correlation of the output level (dangerous failures) and aligns the inputs into the power system. The only question is, at what price and with what losses may transfer energy to such distances. The answer depends on different technologies, which today essentially three: the transmission of alternating current, permanent and superconducting wire. Although such a division is a little bit wrong (superconductor may be alternating and direct current), but from a systemic point of view is legitimate.
However, the technique for transmission of high voltage, in my opinion, is one of the most fabulous-looking. Photo rectifier station at 600 kV.
Traditional power generation from the outset was the way of uniting the power generation using high-voltage power lines with alternating current, when in the 70s up to 750-800-kilovolt transmission line that can transmit 2-3 gigawatts of power. Such transmission has approached the limits of the classical AC power: on the one hand, on the system constraints associated with the complexity of the synchronization of networks many thousands of kilometers and the desire to divide it up into power districts, associated insuring relatively small lines, and on the other hand, because of the rise of reactive power and losses of such a line (associated with the increasing inductance of the line and the capacitive coupling to earth).
Not quite a typical picture of the energy sector in Russia at the time of writing, but usually the flows between the regions do not exceed 1-2 GW.
However, the appearance of the power systems of the 70s and 80s did not require the powerful and distant power lines — a power plant is often easier to push to consumers, and the only exception was then-renewable energy — hydro generation.
Hydroelectric power, specifically the Brazilian project, the Itaipu hydroelectric dam in the mid-80s led to the emergence of a new champion for electricity transmission are many and far — DC transmission line. Power Brazilian link — 2 3150 MW at a voltage of +-600 kV at a distance of 800 km, the project is implemented by the firm ABB. Such power has on the verge is available, AC lines, but the big losses were paid for the project with conversion to direct current.
Itaipu hydroelectric power plant with a capacity of 14 GW is still the second largest hydroelectric power station. Of the energy produced is transmitted via the HVDC link in the district of San Paolo and Rio de Janeiro.
Unlike AC lines, power lines PT free from inductive and capacitive losses (i.e. losses through parasitic capacitive and inductive coupling of the conductor with the surrounding land and water), and originally were widely used mainly for connection to the common power system of large Islands submarine cables, where the loss of the AC line into the water can reach 50-60% capacity. In addition, transmission lines of PT when the same voltage level and the wire cross section that can transmit 15% more power on two wires than AC lines for the three. Problems with isolation of the DC ETL easier — because with alternating current the maximum amplitude of the voltage to 1.41 times more than the current, which is power. Finally, LEP PT does not require synchronization of the generators on the two sides, and thus eliminates many of the problems associated with synchronization remote areas.
Compare AC power lines (AC) and direct current (DC) current. The comparison is a little advertising, because at the same current (say 4000 A), AC lines and 800 kV will have a capacity of 5.5 GW versus 6.4 GW from power lines DC, but with twice as large losses. With the same losses, do the power will be different in 2 times.
The calculation of losses for different variants of power lines, which is intended to be used in the Desertec project.
Of course, there are negatives and significant. First, a constant current in the power system of AC requires rectification on the one hand and "zakreplenie" (i.e. generating a synchronous sine) on the other. When it comes to many gigawatts or hundreds of kilovolts — this is highly nontrivial (and very beautiful!) equipment that costs many hundreds of millions of dollars. In addition, prior to 2010 years power lines PT could only be of the form "point to point", because there were no adequate switches to such voltage and power DC, and therefore for many consumers it was impossible to cut off one of them with a short circuit — just to pay off the entire system. So the main use of powerful lines of PT — connection of the two power districts, where needed large flows. Just a few years ago ABB (one of the three leaders in the creation of the HVDC equipment) managed to create a “hybrid” tirestore-mechanical switch (similar ideas with switch ITER), which is capable of such a job, and now construction of the first high-voltage transmission lines PT “point-to-multipoint” North-East of Angra in India.
The hybrid breaker ABB not sufficiently expressive (and not illuminated), but there is megapafosnyh Hindu video on the Assembly of a mechanical switch, for a voltage of 1200 kV — an impressive machine!
However, the technology PT-energy was developed and became cheaper (largely due to the development of power semiconductors), and to the emergence of gigawatts renewable energy-generation were quite ready in order to begin to connect remote powerful hydroelectric power station and wind farms to consumers. Especially many of these projects have been implemented in recent years in China and India.
But the idea goes further. In many models the possibility of PT-power lines for energy transmission are used to align RES-variability, which is an essential factor in the implementation of 100% renewable energy in large power systems. Moreover, this approach is already being implemented on the example of 1.4 gigawatt link Germany-Norway, designed to compensate for the variability of German wind energy in Norwegian pumped storage power station and the 500 megawatt link Australia-Tasmania needed to maintain power system of Tasmania (mainly hydro) in drought conditions.
Much of the credit for spreading HVDC belongs to the same progress in the cables (as is often the HVDC is offshore), which over the past 15 years has raised the available voltage from 400 to 620 kV
However, further circulation is prevented as the high cost of the transmission lines of similar caliber (for example, the world's largest LEP PT Xinjiang Anhui 10 GW of 3,000 km would cost the Chinese approximately $ 5 billion), and the lack of development of equivalent areas of RES-generation, i.e. the lack of around the major consumers (e.g. Europe or China) are comparable to large consumers at a distance up to 3-5 thousand km.
Including about 30% of the cost of transmission lines PT lines are here such Converter station.
However, what if the technology of the transmission line at the same time cheaper and with fewer losses (which determine the maximum reasonable length?). For example, transmission lines with superconducting cables.
An example of a real superconducting cable for the AMPACITY project. In the center of the former with liquid nitrogen, on it are 3-phase superconducting wire of the ribbons with high temperature superconductor separated by insulation, the outside of the copper screen, another channel with liquid nitrogen, is surrounded by a multilayer screen-vacuum thermal insulation inside the vacuum cavity and the outside protective shell polymer.
Of course, the first superconducting transmission line projects and their economic calculations did not appear today and not yesterday, and in the early 60-ies immediately after the opening of the “industrial” superconductors based on intermetallic compounds of niobium. However, for classic networks without renewable energy such SP transmission was not — and from the perspective of a reasonable power and cost of such power lines, and the point of view of volume of developments required to implement them in practice.
The project of a superconducting cable line of 1966 — 100 GW, 1000 km, with a clear underestimation of the cost of cryogenic parts and voltage converters.
The economy of the superconducting line is determined by essentially two things: the cost of the superconducting cable and energy for cooling. The original idea of using niobium intermetallide tripped on the high cost of cooling with liquid helium: the internal “cold” electrical Assembly must be kept in vacuum (that is not difficult) and advanced to surround a liquid nitrogen cooled screen, otherwise the heat flow at the temperature of 4.2 K will exceed the reasonable capacity of the refrigerators. Such a “sandwich” plus the presence of two costly cooling systems at the time, buried the interest in SP-LEP.
A return to the idea came with the discovery of high temperature conductors and “medium temperature” magnesium diboride MgB2. The cooling temperature of 20 Kelvin (K) for diboride or 70 K (70 K — the temperature of liquid nitrogen is widely utilized, and the cost of this refrigerant is low) for HTSC looks interesting. The first superconductor for today is fundamentally cheaper than the manufactured semiconductor processing methods of HTS-tape.
Three-phase superconducting cable (glands in the cryogenic part in the background) of the draft LIPA in the United States, each with a current of 2400 A and a voltage of 138 kV with a total capacity of 574 MW.
Specific numbers for today are as follows: HTS is the cost of the conductor in 300-400 dollars per kA*m (i.e., meter conduit, which can withstand kiloamps) for liquid nitrogen and 100-130 dollars for 20 K, magnesium diboride, for a temperature of 20 K has a value of 2-10 $ per kA*m (price not well established, and technology), niobate titanium — about $ 1 per kA*m, but for temperature at 4.2 K. For comparison, the aluminum wire transmission lines cost ~5-7 per kA*m copper — 20.
Real heat losses SP cable AMPACITY 1 km long and a capacity of ~40 MW. In terms of power cryocooler and circulation pump, the power required for the operation of the cable, is about 35 kW, or less than 0.1% of the transmitted power.
Of course, the fact that the SP cable is a complex evacuated product, which can be routed just under the ground, adds additional costs, however, where the land under power lines is expensive (e.g. in cities), SP transmission lines are already beginning to emerge, albeit still in the form of pilot projects. Basically, it's the cables from the HTS (as most used), low and medium voltage (10 to 66 kV), currents from 3 to 20 kA. This scheme minimizes the number of intermediate members associated with an increase in voltage in the line (transformers, switches, etc.) Most ambitious and already implemented project of power cable is a project LIPA: three cable length of 650 meters, is designed for transmitting three-phase current with a power of 574 MVA, which is comparable to air power line 330 kV. Commissioning of the most powerful to date of HTS cable line took place on 28 June 2008
An interesting project is implemented AMPACITY in Essen, Germany. Cable medium voltage (10 kV c current 2300 A capacity of 40 MVA) with an integrated superconducting current limiter (is actively developing an interesting technology due to the loss of superconductivity "naturally" to disconnect the cable in the case of overload short circuit) installed inside urban areas. The launch was performed in April 2014, This cable will become a prototype for other projects planned in Germany on the replacement of 110 kV cables transmission line on the superconducting 10 kV cables.
Installation of cable AMPACITY is comparable to drawing a conventional high-voltage cables.
Pilot projects with different superconductors at different values of current and voltage even more, including some made in our country, for example, testing an experimental 30 meter cable with a MgB2 superconductor, cooled by liquid Vodacom. The cable under a constant current of 3500 A and a voltage of 50 kV, created VNIIKP interesting "hybrid scheme" where cooling hydrogen at the same time is a promising method of transport of hydrogen under the idea of "hydrogen energy".
But back to renewable energy. The LUT simulation was aimed at creating a 100% renewable energy generation across the continent, while the cost of electricity had to be less than $ 100 per MW*h. the special Feature of the model is the resulting flows in the tens of gigawatts between European countries. Such power is almost impossible to convey in any way except SP DC transmission line.
Simulation data LUT for the UK require electricity exports, reaching 70 GW in the presence of today links the island's 3.5 GW and extend this value up to 10 GW in the foreseeable future.
And such projects exist. For example Carlo Rubbia, familiar to us from the reactor MYRRHA accelerator driver, is promoting projects based on almost the only manufacturer in the world of strandow of magnesium diboride — on the idea of a cryostat with a diameter of 40 cm (however, it's quite difficult for transportation and stacking on land diameter) can accommodate 2 cables with a current of 20 kA and a voltage of +-250 kV, i.e. total capacity of 10 GW, and in this cryostat can accommodate 4 Explorer = 20 GW, which is close to the desired model of the LUT, and in contrast to conventional high-voltage direct-current lines, there is still a large margin to increase power. The cost of power for refrigeration and pumping hydrogen amount to ~10 MW per 100 km, or 300 MW at 3,000 km — about three times less than for the most advanced high-voltage direct-current lines.
Offer Rubbia 10-gigawatt cable transmission lines. A giant pipe size for liquid hydrogen is needed in order to reduce hydraulic resistance and to be able to put the intermediate cristinzio at most 100 km. There is a problem with maintaining vacuum on a pipe (distributed ion vacuum pump — not the wisest decision here, IMHO)
If you continue to increase the size of the cryostat to values typical for natural gas pipelines (1200 mm) to fit inside 6-8 conductors 20 kA and 620 kV (peak mastered on today voltage cables), the power of this “pipe” will amount to 100 GWh, which exceeds the power transferred by gas and oil pipelines (the most powerful of which transmit the equivalent of 85 GWh heat). The main problem may be connecting such highway to existing networks, however, the fact that the technology is almost available.
It is interesting to estimate the cost of such line.
To dominate, obviously the construction part. For example, the laying of 800 km 4 HVDC cables in the German project Sudlink cost ~8-10 billion euros (this is known because the project has risen from 5 to 15 billion after the transition from overhead lines to cable). The installation cost 10-12 million euros per km approximately 4-4. 5 times higher than the average cost of natural gas pipelines, according to this study.
In principle, nothing prevents to use a similar technique for laying heavy duty power lines, however, the main difficulties here are in the terminal stations and connection to existing networks.
If you take something between a gas and cables (i.e. 6-8 million euros per km), the cost of the superconductor is likely to be lost in the cost of construction: 100-gigawatt line the value of SP will be ~ $ 0.6 million per 1 km, if you take the SP cost 2$ per kA*m.
An interesting dilemma emerges: SP “megamusical” are several times more expensive than gas lines with comparable power (remember that it's all in the future. Today the situation is even worse — you need to recoup R & d SP-LEP), and this is why pipelines are being built, but not SP-LEP. However, the growth of renewable energy, this technology can be attractive to obtain a rapid development. The project Sudlink, probably would run in the SP cable, if the technology would be ready. published
P. S. And remember, only by changing their consumption — together we change the world! ©
Source: //geektimes.ru/post/288386/
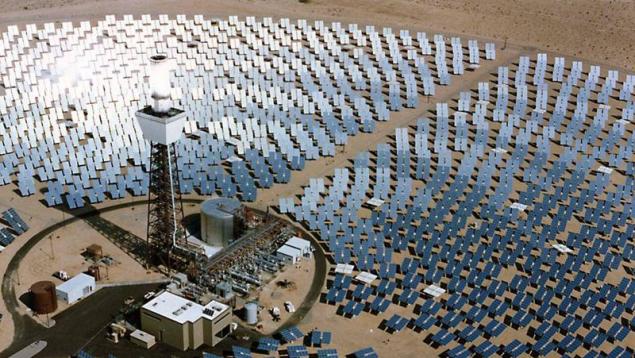
SES of this kind were to become the main European renewable energy industry.
The project lasted about 10 years, and was then abandoned by the companies-founders, as reality European green energy was completely different and more prosaic — Chinese photovoltaics and ground vetrogeneratory placed in Europe itself, and the idea is to pull the energy of the line through Libya and Syria — are too optimistic.
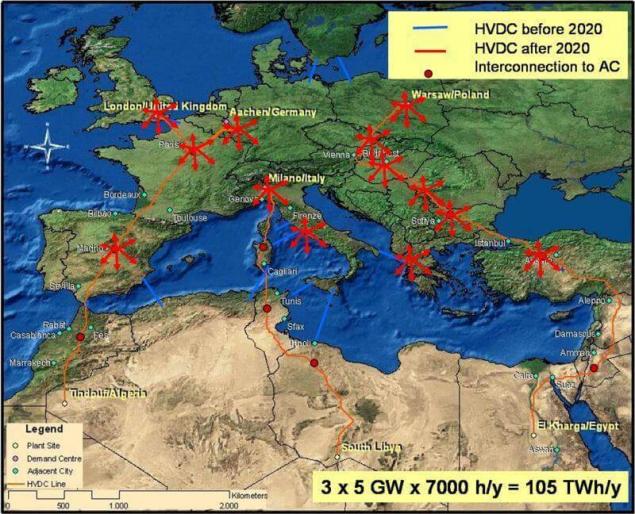
Planned in the framework of the desertec transmission lines: three main areas with a capacity of 3x10 gigawatts (in the picture one of the weaker versions 3x5) and several submarine cables.
However, powerful lines appeared in the "desertec" is no accident (funny, incidentally, that the area of land under power lines in the project was more land under the SES) is one of the key technologies that could enable renewable energy generation to grow to an overwhelming proportion, and Vice versa: in the absence of the technology of transmission of energy over long distances RES, quite possibly, doomed to no more than a fraction of 30-40% in the energy sector of Europe.
Mutual synergy transcontinental transmission lines and renewable energy is quite well visible in the models (for example, a giant model of the LUT, and also model Vyacheslav Laktyushina): the Union of many areas of wind generation, disposed in 1-2-3 thousands of kilometers from each other, destroys the cross-correlation of the output level (dangerous failures) and aligns the inputs into the power system. The only question is, at what price and with what losses may transfer energy to such distances. The answer depends on different technologies, which today essentially three: the transmission of alternating current, permanent and superconducting wire. Although such a division is a little bit wrong (superconductor may be alternating and direct current), but from a systemic point of view is legitimate.
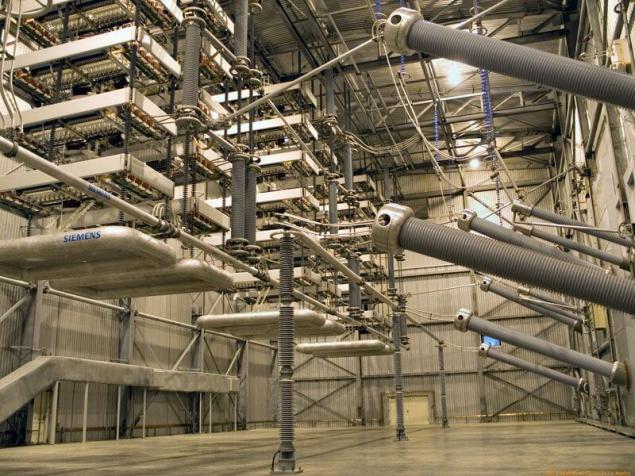
However, the technique for transmission of high voltage, in my opinion, is one of the most fabulous-looking. Photo rectifier station at 600 kV.
Traditional power generation from the outset was the way of uniting the power generation using high-voltage power lines with alternating current, when in the 70s up to 750-800-kilovolt transmission line that can transmit 2-3 gigawatts of power. Such transmission has approached the limits of the classical AC power: on the one hand, on the system constraints associated with the complexity of the synchronization of networks many thousands of kilometers and the desire to divide it up into power districts, associated insuring relatively small lines, and on the other hand, because of the rise of reactive power and losses of such a line (associated with the increasing inductance of the line and the capacitive coupling to earth).
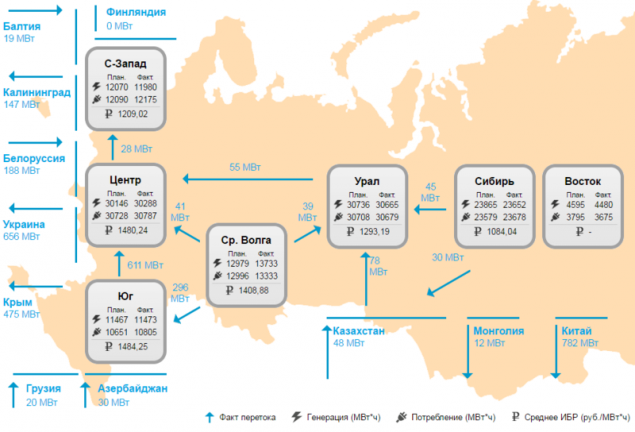
Not quite a typical picture of the energy sector in Russia at the time of writing, but usually the flows between the regions do not exceed 1-2 GW.
However, the appearance of the power systems of the 70s and 80s did not require the powerful and distant power lines — a power plant is often easier to push to consumers, and the only exception was then-renewable energy — hydro generation.
Hydroelectric power, specifically the Brazilian project, the Itaipu hydroelectric dam in the mid-80s led to the emergence of a new champion for electricity transmission are many and far — DC transmission line. Power Brazilian link — 2 3150 MW at a voltage of +-600 kV at a distance of 800 km, the project is implemented by the firm ABB. Such power has on the verge is available, AC lines, but the big losses were paid for the project with conversion to direct current.
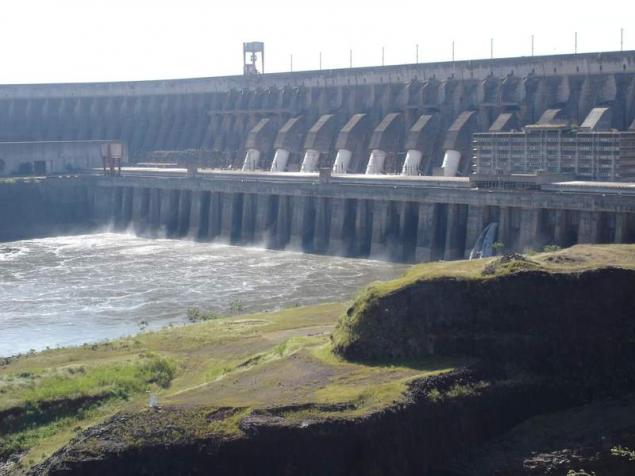
Itaipu hydroelectric power plant with a capacity of 14 GW is still the second largest hydroelectric power station. Of the energy produced is transmitted via the HVDC link in the district of San Paolo and Rio de Janeiro.
Unlike AC lines, power lines PT free from inductive and capacitive losses (i.e. losses through parasitic capacitive and inductive coupling of the conductor with the surrounding land and water), and originally were widely used mainly for connection to the common power system of large Islands submarine cables, where the loss of the AC line into the water can reach 50-60% capacity. In addition, transmission lines of PT when the same voltage level and the wire cross section that can transmit 15% more power on two wires than AC lines for the three. Problems with isolation of the DC ETL easier — because with alternating current the maximum amplitude of the voltage to 1.41 times more than the current, which is power. Finally, LEP PT does not require synchronization of the generators on the two sides, and thus eliminates many of the problems associated with synchronization remote areas.
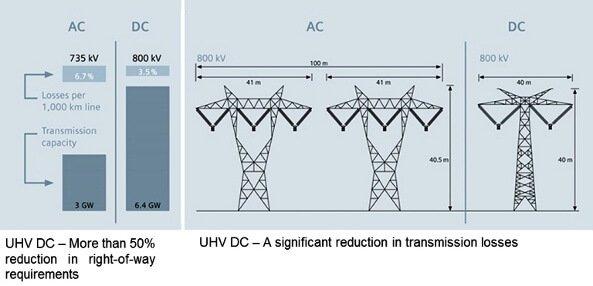
Compare AC power lines (AC) and direct current (DC) current. The comparison is a little advertising, because at the same current (say 4000 A), AC lines and 800 kV will have a capacity of 5.5 GW versus 6.4 GW from power lines DC, but with twice as large losses. With the same losses, do the power will be different in 2 times.
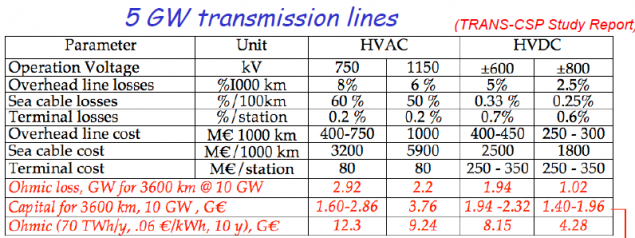
The calculation of losses for different variants of power lines, which is intended to be used in the Desertec project.
Of course, there are negatives and significant. First, a constant current in the power system of AC requires rectification on the one hand and "zakreplenie" (i.e. generating a synchronous sine) on the other. When it comes to many gigawatts or hundreds of kilovolts — this is highly nontrivial (and very beautiful!) equipment that costs many hundreds of millions of dollars. In addition, prior to 2010 years power lines PT could only be of the form "point to point", because there were no adequate switches to such voltage and power DC, and therefore for many consumers it was impossible to cut off one of them with a short circuit — just to pay off the entire system. So the main use of powerful lines of PT — connection of the two power districts, where needed large flows. Just a few years ago ABB (one of the three leaders in the creation of the HVDC equipment) managed to create a “hybrid” tirestore-mechanical switch (similar ideas with switch ITER), which is capable of such a job, and now construction of the first high-voltage transmission lines PT “point-to-multipoint” North-East of Angra in India.
The hybrid breaker ABB not sufficiently expressive (and not illuminated), but there is megapafosnyh Hindu video on the Assembly of a mechanical switch, for a voltage of 1200 kV — an impressive machine!
However, the technology PT-energy was developed and became cheaper (largely due to the development of power semiconductors), and to the emergence of gigawatts renewable energy-generation were quite ready in order to begin to connect remote powerful hydroelectric power station and wind farms to consumers. Especially many of these projects have been implemented in recent years in China and India.
But the idea goes further. In many models the possibility of PT-power lines for energy transmission are used to align RES-variability, which is an essential factor in the implementation of 100% renewable energy in large power systems. Moreover, this approach is already being implemented on the example of 1.4 gigawatt link Germany-Norway, designed to compensate for the variability of German wind energy in Norwegian pumped storage power station and the 500 megawatt link Australia-Tasmania needed to maintain power system of Tasmania (mainly hydro) in drought conditions.
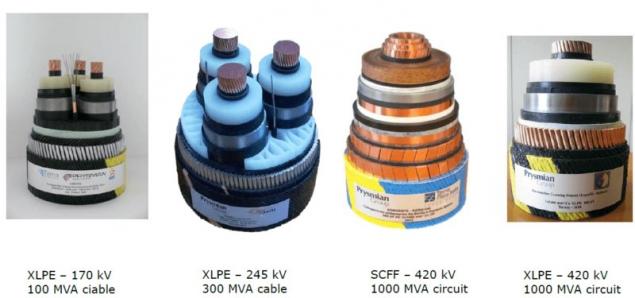
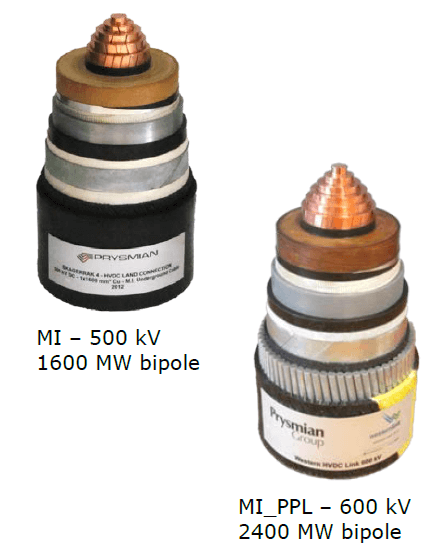
Much of the credit for spreading HVDC belongs to the same progress in the cables (as is often the HVDC is offshore), which over the past 15 years has raised the available voltage from 400 to 620 kV
However, further circulation is prevented as the high cost of the transmission lines of similar caliber (for example, the world's largest LEP PT Xinjiang Anhui 10 GW of 3,000 km would cost the Chinese approximately $ 5 billion), and the lack of development of equivalent areas of RES-generation, i.e. the lack of around the major consumers (e.g. Europe or China) are comparable to large consumers at a distance up to 3-5 thousand km.
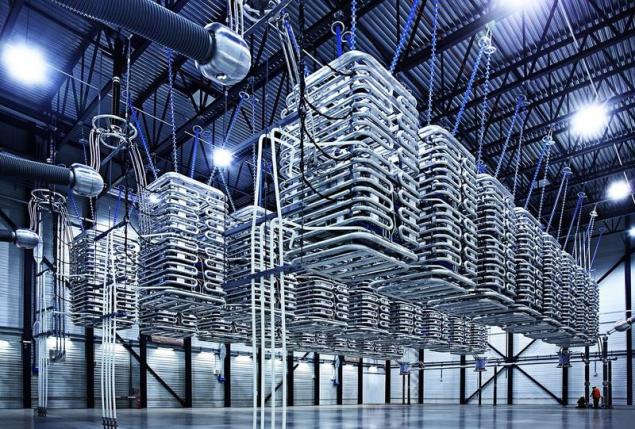
Including about 30% of the cost of transmission lines PT lines are here such Converter station.
However, what if the technology of the transmission line at the same time cheaper and with fewer losses (which determine the maximum reasonable length?). For example, transmission lines with superconducting cables.
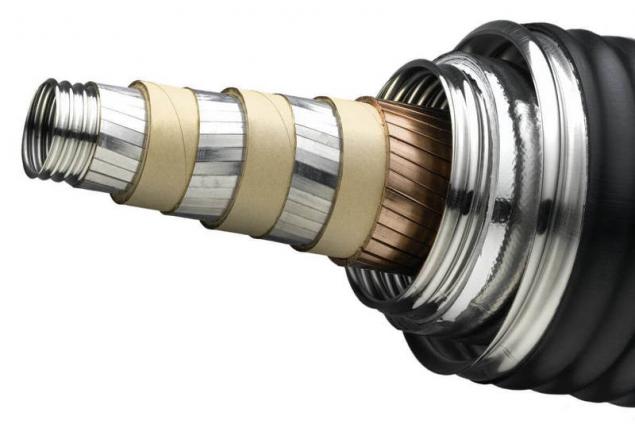
An example of a real superconducting cable for the AMPACITY project. In the center of the former with liquid nitrogen, on it are 3-phase superconducting wire of the ribbons with high temperature superconductor separated by insulation, the outside of the copper screen, another channel with liquid nitrogen, is surrounded by a multilayer screen-vacuum thermal insulation inside the vacuum cavity and the outside protective shell polymer.
Of course, the first superconducting transmission line projects and their economic calculations did not appear today and not yesterday, and in the early 60-ies immediately after the opening of the “industrial” superconductors based on intermetallic compounds of niobium. However, for classic networks without renewable energy such SP transmission was not — and from the perspective of a reasonable power and cost of such power lines, and the point of view of volume of developments required to implement them in practice.
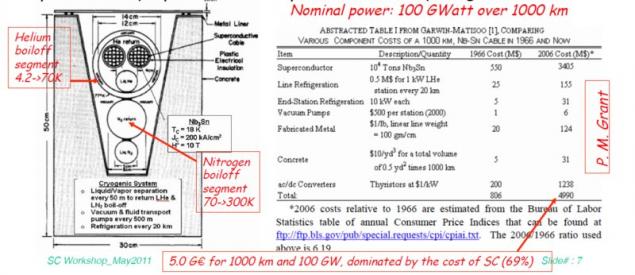
The project of a superconducting cable line of 1966 — 100 GW, 1000 km, with a clear underestimation of the cost of cryogenic parts and voltage converters.
The economy of the superconducting line is determined by essentially two things: the cost of the superconducting cable and energy for cooling. The original idea of using niobium intermetallide tripped on the high cost of cooling with liquid helium: the internal “cold” electrical Assembly must be kept in vacuum (that is not difficult) and advanced to surround a liquid nitrogen cooled screen, otherwise the heat flow at the temperature of 4.2 K will exceed the reasonable capacity of the refrigerators. Such a “sandwich” plus the presence of two costly cooling systems at the time, buried the interest in SP-LEP.
A return to the idea came with the discovery of high temperature conductors and “medium temperature” magnesium diboride MgB2. The cooling temperature of 20 Kelvin (K) for diboride or 70 K (70 K — the temperature of liquid nitrogen is widely utilized, and the cost of this refrigerant is low) for HTSC looks interesting. The first superconductor for today is fundamentally cheaper than the manufactured semiconductor processing methods of HTS-tape.
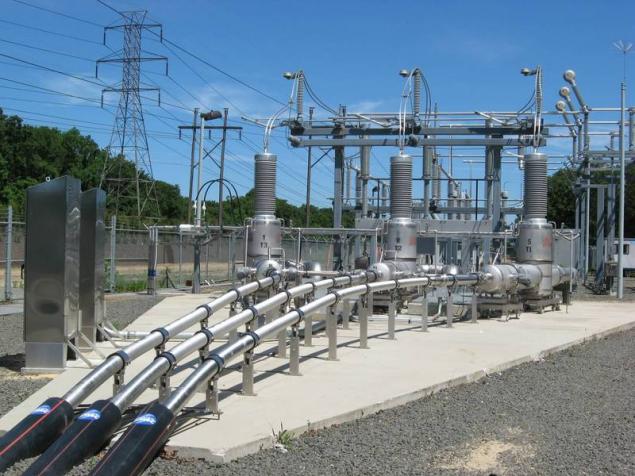
Three-phase superconducting cable (glands in the cryogenic part in the background) of the draft LIPA in the United States, each with a current of 2400 A and a voltage of 138 kV with a total capacity of 574 MW.
Specific numbers for today are as follows: HTS is the cost of the conductor in 300-400 dollars per kA*m (i.e., meter conduit, which can withstand kiloamps) for liquid nitrogen and 100-130 dollars for 20 K, magnesium diboride, for a temperature of 20 K has a value of 2-10 $ per kA*m (price not well established, and technology), niobate titanium — about $ 1 per kA*m, but for temperature at 4.2 K. For comparison, the aluminum wire transmission lines cost ~5-7 per kA*m copper — 20.
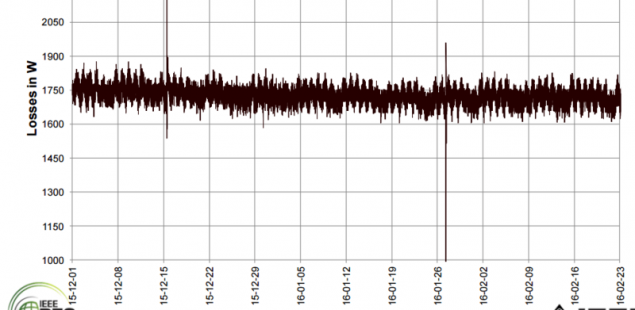
Real heat losses SP cable AMPACITY 1 km long and a capacity of ~40 MW. In terms of power cryocooler and circulation pump, the power required for the operation of the cable, is about 35 kW, or less than 0.1% of the transmitted power.
Of course, the fact that the SP cable is a complex evacuated product, which can be routed just under the ground, adds additional costs, however, where the land under power lines is expensive (e.g. in cities), SP transmission lines are already beginning to emerge, albeit still in the form of pilot projects. Basically, it's the cables from the HTS (as most used), low and medium voltage (10 to 66 kV), currents from 3 to 20 kA. This scheme minimizes the number of intermediate members associated with an increase in voltage in the line (transformers, switches, etc.) Most ambitious and already implemented project of power cable is a project LIPA: three cable length of 650 meters, is designed for transmitting three-phase current with a power of 574 MVA, which is comparable to air power line 330 kV. Commissioning of the most powerful to date of HTS cable line took place on 28 June 2008
An interesting project is implemented AMPACITY in Essen, Germany. Cable medium voltage (10 kV c current 2300 A capacity of 40 MVA) with an integrated superconducting current limiter (is actively developing an interesting technology due to the loss of superconductivity "naturally" to disconnect the cable in the case of overload short circuit) installed inside urban areas. The launch was performed in April 2014, This cable will become a prototype for other projects planned in Germany on the replacement of 110 kV cables transmission line on the superconducting 10 kV cables.
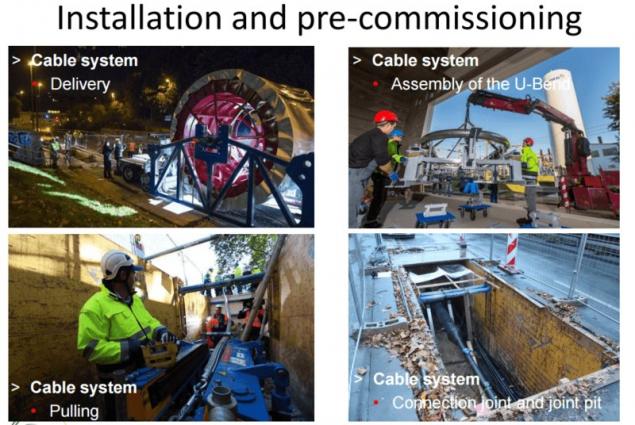
Installation of cable AMPACITY is comparable to drawing a conventional high-voltage cables.
Pilot projects with different superconductors at different values of current and voltage even more, including some made in our country, for example, testing an experimental 30 meter cable with a MgB2 superconductor, cooled by liquid Vodacom. The cable under a constant current of 3500 A and a voltage of 50 kV, created VNIIKP interesting "hybrid scheme" where cooling hydrogen at the same time is a promising method of transport of hydrogen under the idea of "hydrogen energy".
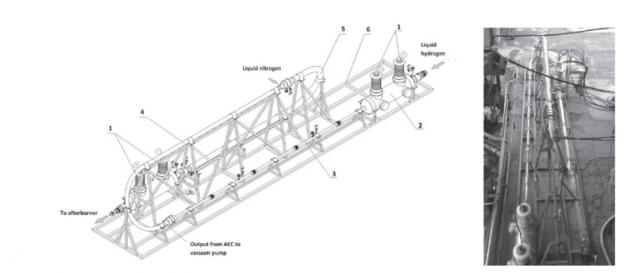
But back to renewable energy. The LUT simulation was aimed at creating a 100% renewable energy generation across the continent, while the cost of electricity had to be less than $ 100 per MW*h. the special Feature of the model is the resulting flows in the tens of gigawatts between European countries. Such power is almost impossible to convey in any way except SP DC transmission line.
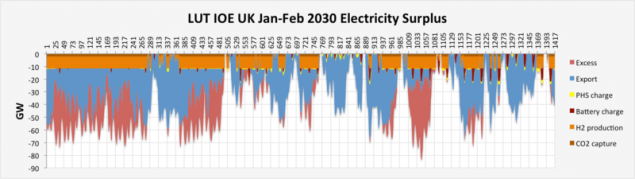
Simulation data LUT for the UK require electricity exports, reaching 70 GW in the presence of today links the island's 3.5 GW and extend this value up to 10 GW in the foreseeable future.
And such projects exist. For example Carlo Rubbia, familiar to us from the reactor MYRRHA accelerator driver, is promoting projects based on almost the only manufacturer in the world of strandow of magnesium diboride — on the idea of a cryostat with a diameter of 40 cm (however, it's quite difficult for transportation and stacking on land diameter) can accommodate 2 cables with a current of 20 kA and a voltage of +-250 kV, i.e. total capacity of 10 GW, and in this cryostat can accommodate 4 Explorer = 20 GW, which is close to the desired model of the LUT, and in contrast to conventional high-voltage direct-current lines, there is still a large margin to increase power. The cost of power for refrigeration and pumping hydrogen amount to ~10 MW per 100 km, or 300 MW at 3,000 km — about three times less than for the most advanced high-voltage direct-current lines.
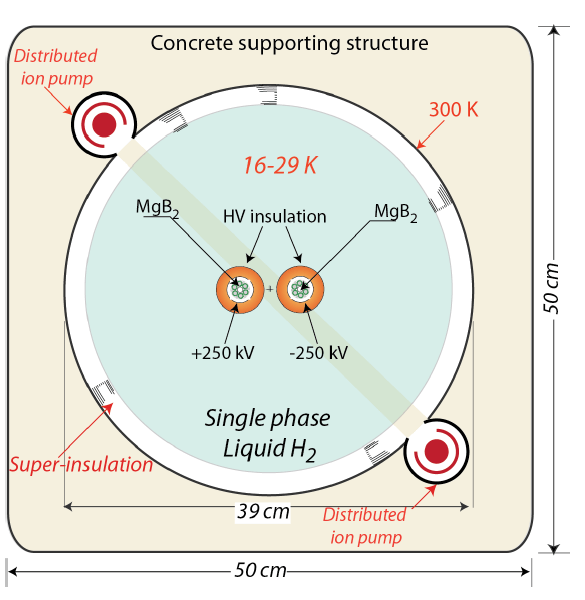
Offer Rubbia 10-gigawatt cable transmission lines. A giant pipe size for liquid hydrogen is needed in order to reduce hydraulic resistance and to be able to put the intermediate cristinzio at most 100 km. There is a problem with maintaining vacuum on a pipe (distributed ion vacuum pump — not the wisest decision here, IMHO)
If you continue to increase the size of the cryostat to values typical for natural gas pipelines (1200 mm) to fit inside 6-8 conductors 20 kA and 620 kV (peak mastered on today voltage cables), the power of this “pipe” will amount to 100 GWh, which exceeds the power transferred by gas and oil pipelines (the most powerful of which transmit the equivalent of 85 GWh heat). The main problem may be connecting such highway to existing networks, however, the fact that the technology is almost available.
It is interesting to estimate the cost of such line.
To dominate, obviously the construction part. For example, the laying of 800 km 4 HVDC cables in the German project Sudlink cost ~8-10 billion euros (this is known because the project has risen from 5 to 15 billion after the transition from overhead lines to cable). The installation cost 10-12 million euros per km approximately 4-4. 5 times higher than the average cost of natural gas pipelines, according to this study.
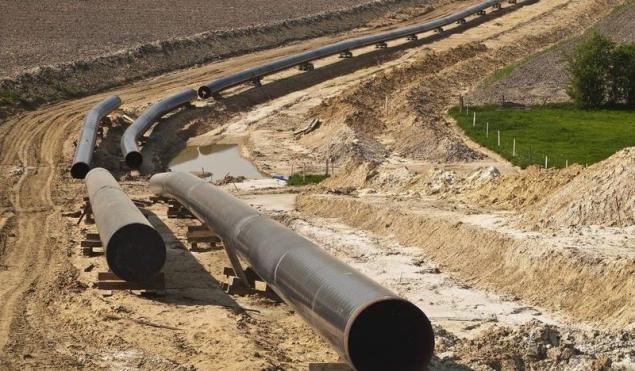
In principle, nothing prevents to use a similar technique for laying heavy duty power lines, however, the main difficulties here are in the terminal stations and connection to existing networks.
If you take something between a gas and cables (i.e. 6-8 million euros per km), the cost of the superconductor is likely to be lost in the cost of construction: 100-gigawatt line the value of SP will be ~ $ 0.6 million per 1 km, if you take the SP cost 2$ per kA*m.
An interesting dilemma emerges: SP “megamusical” are several times more expensive than gas lines with comparable power (remember that it's all in the future. Today the situation is even worse — you need to recoup R & d SP-LEP), and this is why pipelines are being built, but not SP-LEP. However, the growth of renewable energy, this technology can be attractive to obtain a rapid development. The project Sudlink, probably would run in the SP cable, if the technology would be ready. published
P. S. And remember, only by changing their consumption — together we change the world! ©
Source: //geektimes.ru/post/288386/
Method of production of 10 terawatts of energy from sunlight by 2030
The program TrussFab allows you to build anything from plastic bottles