102
Can the LHC test string theory?
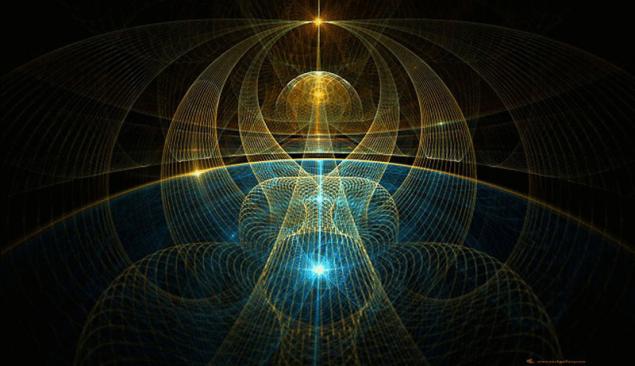
String theory, the ultimate theory, is the mythical arbiter of truth, heralding the end of science. Praised for its beauty and elegance, string theory, like no theory before it, attracts the attention of not only physicists but also the public. String theorists have boldly gone where no human foot has yet gone: explore marvelous new worlds, 10^500 or so, most of which are uninhabitable. They brought back extra dimensions, supersymmetry, and the promise of reconciling Einstein’s general theory of relativity with the Standard Model. More than that, not just a promise, but a fact: they say they did it. The only thing left is to test them.
The Large Hadron Collider (LHC) operates in a range of energies that have long remained inaccessible to humans, and after a short break again dives into the unknown. The new launch is an unprecedented opportunity to study the structure of elementary matter at the smallest distances, down to one thousandth of a femtometer. Will this help to confirm the correctness of string theorists that everything is made of strings, that everywhere invisible loops of quantum gravity?
As is often the case in science, the answer is: it depends on what you ask for.
String theory began with an attempt to describe the strong nuclear force in the 80s. And although it was quickly overtaken by the more successful theory of quantum chromodynamics (quarks, gluons, etc.), strings still play an important role in describing strong interactions. The “Lund string model,” named after the Swedish city where it was conceived, describes the interaction of gluons using streaming tubes called strings. Stretching them and the strings will crumble with a shower of secondary particles. This so-called “string fragmentation” is an integral part of many computer models that are required to produce accurate predictions of LHC scattering events.
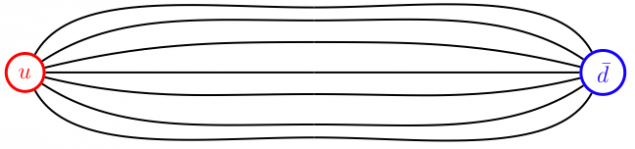
Lund’s string model has long been used, and no one expects the LHC data to tell anything new about it. Aside from dealing with strings, this model has little in common with string theory, which we know and love. The Lund model is an approximation that complements the standard model of particle interaction; it has nothing to do with combining gravity and the standard model, it does not use extra dimensions and supersymmetry.
So what about the ultimate theory that postulates that all of our particles are strings with a certain vibration, theories with extra dimensions and supersymmetry, and endless debates about its superiority over loop quantum gravity?
Strings can be excited to higher modes, a prediction characteristic of string theory and in principle observable. The energy required for these excitations depends on the radius of the extra dimensions of string theory: the smaller the radius, the greater the energy required to excite the strings. The most natural scenario places the radius of extra dimensions within the string: it's about 10^19 GeV. In this case, string theory is hopeless outside the search radius of the LHC, which is gaining a maximum of 104 GeV, even taking into account the latest upgrade.
In the event that the extra dimensions of string theory are quite large and within the range detectable at the LHC, they may be quite noticeable. The production of tiny black holes is one prediction. This process is possible because the extra dimensions make gravity at short distances stronger than the three-dimensional theory of general relativity predicts. For the same reason, the production of gravitons, quanta of gravity, could become possible at the LHC if the extra dimensions are large. These phenomena can lead to certain observed effects that have been calculated in good detail.
The extra dimensions alone do not tell us that string theory is correct, as it is only one ingredient in a complete theory. However, if we can find evidence of extra dimensions, it would be a powerful argument for string theory and the emergence of a true string phenomenology that could manifest in string balls or something else. Additional measurements are the best way to test string theory at the LHC.
Physicists have carefully tested previous LHC launches for the appearance of black holes or gravitons that could have their say in favor of extra dimensions. They didn't find anything. It remains possible that these phenomena will show themselves at a higher energy investment, but there are fewer and fewer scientists who believe this.
Supersymmetry is another consequence of string theory, which, if discovered, would be a strong word for string theory, but not a definitive confirmation of string theory. Supersymmetry predicts that all particles have pairs. Since we have not found supersymmetric partners of the known particles, the supersymmetry must be broken in such a way that the partner particles are too heavy to be observed.
Before the LHC began operations, there were widespread arguments that partner particles would be available during the first launches. They were based more honorably on the technical beauty of the idea, the hypothesis itself. But they never found anything. As in the case of additional measurements, there is hope that further work of the LHC will still be able to reveal traces of unknown particles.
You probably want to know what the chances are that the LHC will support string theory? A counter-question: If you're hooked on Xyusha, and she says she'll be free after work, around eight in the evening, but doesn't show up at ten, what are the chances she'll come at 10:05? Here. While additional dimensions never promised to come, hope is for the worst.
String theorists are no longer waiting for a final theory. They see string theory as a tool that enables complex calculations in the standard model, yielding previously unknown data. And they've got work to do.
You see, knowledge of the Standard Model and theory on paper are one thing. Being able to actually solve equations that describe specific systems is quite another thing. The methods commonly used by theoretical physicists do not work well in situations where many particles interact strongly, such as the quark-gluon plasma created by collisions in large atomic nuclei (heavy ions) at the LHC. But in such situations, the methods developed in string theory offer new solutions.
String theory helps to describe quark-gluon plasma using so-called gauge-gravitational duality, which manifests as a particular manifestation of a more general duality in string theory. Calibration-gravitational duality can be used to map complex and tightly coupled systems as a completely different system that is much easier to work with. In fact, this duality has already been used to predict quark-gluon plasma at the LHC, in particular the energy loss of particles passing through quark-gluon plasma.
Scientific history is moving in a spiral. String theory is again used to describe nuclear interactions.
Unfortunately, the predictions of string theorists do not agree well with the data. The quark-gluon plasma was not as strongly bound as thought, so it went beyond the good work of gauge-gravity duality. So the LHC actually tested string theory.
In the next heavy ion launch, which is likely to take place later this year, more data will appear that will allow physicists to better study quark-gluon plasma. The situation does not stand out as clearly as it might seem at the moment, but only because in the physics of heavy ions it is difficult to distinguish anything clearly. So who knows, maybe the gauge-gravitational duality will get a second wind.
Could using string theory to describe strongly bound states of matter say anything about it as a candidate for a theory of everything? At first glance, no. These are completely different problems: different systems, different tests, different conclusions. However, many string theorists believe that since string theory is a theory that can describe quantum gravity, it suggests that string theory would be the correct theory to describe the whole of nature. The conclusion is illogical, but scientists do not give up and believe that string theory will find confirmation in some experiment. If not at the LHC, then somewhere else.
As romance novels teach us, while you dream of a supersymmetrical princess, your destiny can live next door to you, in the form of a girl who helps you solve complex problems. Maybe physicists will someday establish a stable relationship with string theory when reality finally supersedes dreams. And then the LHC will be able to test the strength of the new relationship and how serious this new hobby is. published
P.S. And remember, just changing our consumption – together we change the world!
Source: hi-news.ru